Space: The Microbial Frontier
By Olaf Olson and Tiberius Dobni
As humanity looks towards the future of interplanetary space missions, the understanding of microbiology is of utmost importance. If there are no considerations towards microbiology in space, a virus could lead towards hazardous conditions for an astronaut. Further understandings of the effects of space on microbiology will give us more insight on the probability of life on other planets and in between. This page provides details of what is known about microbial life in spacecraft, how microorganisms could survive in space and harsh extraterrestrial planets and the theory of panspermia which asks the pertinent question of whether life originated elsewhere in the universe. With this information we are left with a question—How do we know that life can exist in space?
History
As we begin to probe the question of how life can survive in space, it is helpful to start with an overview of its history. Below is a timeline of the discoveries, ideas, and observations behind microbial biology in space.
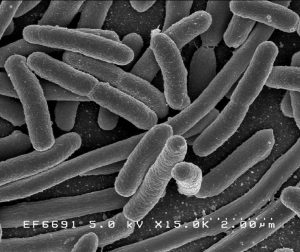
Figure 1– Scanned electron micrograph of Escherichia coli, Credit: Rocky Mountain Laboratories,Source: https://upload.wikimedia.org/wikipedia/commons/thumb/3/32/EscherichiaColi_NIAID.jpg/913px-EscherichiaColi_NIAID.jpg
1908- Svante. A. Arrhenius proposes the theory of panspermia, which entailed that terrestrial beings had originated from beings developed on other planets far from Earth. The theory had been an idea tossed around before, but Arrhenius proposed that microorganisms traveled by means of an acceleration by stellar radiation pressure. It must be noted that the scientific hypothesis explained by astrobiologists does not refer to microorganisms traveling in meteorites and eventually colonizing Earth, but it implies that biological materials from meteorites and comets that crashed in a specific moment of Earth’s evolution and enriched the primordial terrestrial chemistry to create life.1
1960- USSR launches E. coli, Arcobacter, Aerogenes, and Staphylococcus within an unmanned space satellite. Their findings revealed that microgravity of space does not have an effect on the life of a microorganism.2
1967- NASA deploys a satellite with various biological specimens to observe the impact of microgravity in the span of 45 hours. The result found that E. Coli and S. Typhimurium had increased in their population density.2
1972- Apollo 16 and 17 Bacillus subtilis was propagated and the results found that microgravity did not have an effect on spore formation. However, later assessment revealed that the colony forming ability of B. subtilis found that the spores had been reduced among spaceflight samples.2
These early observations grew concerns of microorganisms that responded to the environments of spaceflight. A change in antibiotic resistance (thickening of the cell wall) in E. coli and S. aureus were observed after returning from space.
1995- The term “astrobiology” is coined by Wesley Huntress to describe the expanding studies of the origin, evolution, distribution, and future of life in the universe.3
Late 1990s- In the late 1990s, NASA joined the Russian space program in its evaluation of the microbial activity aboard Mir.4
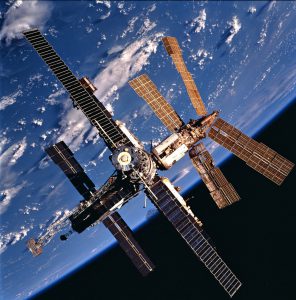
Figure 2– View of Russian Space Station Mir, Credit: NASA, Source: https://upload.wikimedia.org/wikipedia/commons/e/e0/STS86_Mir_Station.jpg
2006- S. Typhimurium among many other microorganisms were launched aboard the Space Shuttle Atlantis. These microorganisms were inspected the disease-causing potential. Mice infected with the disease revealed a decreased time of death/lethal dose and an increased percent mortality. This was a first report that clarified an increased bacterial virulence because of growth in the spaceflight microgravity environment.2
2006-2010- Further investigations of the increased virulence of bacteria revealed that prior findings aboard the Space Shuttle Atlantis were repeated and confirmed.2
2015- In the Japanese Module “Kibo”, sampling was performed to understand the growth of bacteria. Sites of samples were an incubator, door of the inside of an incubator, air intake, air diffuser, and a handrail. Most of the samples found that the bacteria came from the human microbiota, thus suggesting astronauts transferred bacteria to the surfaces. Microbial life may change with the prolonged stay of astronauts, so observations are continual.5
Space Station Observations
The timeline provided displays observations on microbial life that has been made by various space agencies. We will now be looking at the adverse and beneficial effects towards the growth of microbes in the confines of space shuttles through various studies performed on the International Space Station. (ISS)
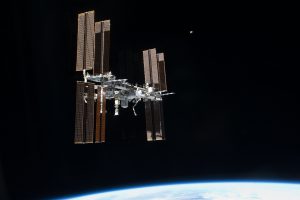
Figure 3– International Space Station as viewed from space shuttle Atlantis 2011, Credit: NASA, Source: https://www.jpl.nasa.gov/images/iss/20170627/ISS20170627.jpg
NASA’s Astronauts set a high standard for cleanliness and stringent monitoring regimes to lower the risks on health and performance.6 With constant monitoring, there is a bountiful amount of information that is provided for analysis. One of the many observations that NASA has found is that microgravity plays an important factor in how it can play a profound effect on matter. Through a period of months, an astronaut will observe adverse effects on their bone and muscle density due to microgravity. Flames are round, and crystals are more perfected without the interference of Earthbound gravity.7 As well, microbial life is profoundly changed, and great caution must be taken when ensuring the cleanliness of a space shuttle.
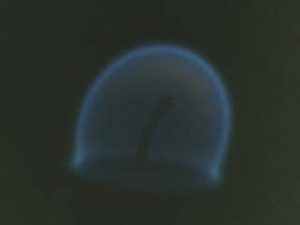
Figure 4– Candle flame in microgravity, Credit: NASA, Source: https://upload.wikimedia.org/wikipedia/commons/8/89/Candle_Flame_in_Microgravity.jpg
These changes of microbial life are detailed in the Russian space shuttle Mir. A series of unfortunate events took place aboard the spacecraft. As expected, the astronauts were rigorous in their scheduled cleaning of all surfaces. However, when the astronauts viewed a rarely accessed service panel they found a brown basketball-sized globule of bacteria floating around and patches of mold inside panels near wiring. Many other globules were found behind other panels. The temperature behind the panels was perfect for the growth of bacteria.8 Later, it was discovered that even more colonies of organisms living in the rubber gaskets around windows, on the components of space suits, cable insulation, and tubing, on the insulation of copper wires, and on communications devices.4 Not only is this an extreme hazard for health, but the damages done to the spacecraft structure could be enormous if it is not quickly eradicated.
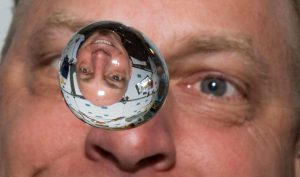
Figure 5– Clayton Anderson, retired astronaut, viewing a more fortunate globule of water. Credit: NASA, Source: https://upload.wikimedia.org/wikipedia/commons/b/b8/Clayton_Anderson_zero_g.jpg
This implies that profound changes happen to microbial life in a confined space shuttle setting. One must understand that the warmth and without human interference, bacteria colonies garner strength in numbers to overwhelm its environment. It must be stressed that physical properties behave extremely differently in a microgravity setting. Such is the case with microorganisms, as they have shown an increase in resistance to physiological and sentimental changes, along with increased virulence. This poses an enormous risk to astronauts that dare to stay in a confined space shuttle without taking into consideration the implications of microbial life.9
Along with the risk of microbes of impacting the success of an astronaut’s work, one must consider the implications on general biology in a microgravity environment. Astronauts are already stressed as they are confined to small spaces, microgravity also leads to a reduction of thirst, atrophy of the muscles, as well as lessened activation of blood circulation and muscle stimulation.10 Due to these issues, an astronaut must be very cautious when considering his or her health. To lower the threat of bacteria being hazardous to an astronaut’s life, monitoring the presence of bacteria is greatly important.
Due to the slow process of culturing bacteria, a faster method of diagnosing the presence of bacteria or fungi on surfaces has been created. Astronauts take a swab of surfaces, mix swabbed material in liquid form to the Lab-on-a-Chip Application Development-Portable Test System (LOCAD-PTS) and within fifteen minutes the results will be displayed on the screen. This type of technology is simply the beginning of quickly monitoring biology in space and one day this could potentially give us the technology for immediate diagnoses of illnesses using blood and saliva tissue.11
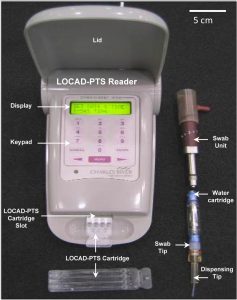
Figure 6– LOCAD-PTS Reader, Credit: NASA, Source: https://upload.wikimedia.org/wikipedia/commons/a/ae/LOCAD-PTS.jpg
Along with microgravity, radiation from the sun and other stars is a factor that is unfortunately understudied and mutations from radiation to the phenotype of an organism is not adequately understood. We do know that direct sunlight kills most microbes that cause airborne infections, but this cannot eliminate anything that is hidden behind shadows crevices or layers of dirt. Microorganisms that leave Earth can become more virulent or more dormant—the probability of what happens is very unpredictable.12
NASA is struggling to understand the adaptability of microorganisms in a space environment that harbors life. To make things more problematic for NASA, a species of bacteria B. pumilus (SAFR-032) has evaded all sterilization techniques. The spores of the bacteria have evolved to be uniquely adaptive in where they build layers of cells to protect their DNA making it resistant to radiation.13 An experiment on the ISS exposed SAFR-032 to an amount of radiation that increased the chance of genetic mutations. This led to increased antibiotic resistance, a frightening aspect to consider if these conditions were met on an actual space mission. SAFR-032 isn’t likely to make astronauts sick, but it must be stressed that it is important to be able to control the bacteria, especially if natural conditions of space aren’t going to kill them off.14
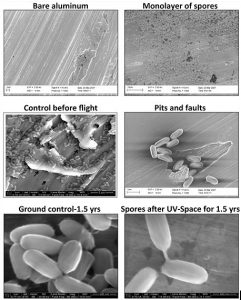
Figure 7– Bottom two pictures, B. pumilus, before and after living in a space environment, Credit: P. Vaishampayan, Source: https://www.nasa.gov/sites/default/files/bacillus.jpg
The importance of this study has most relevance considering bacterial life on Earth. By understanding how microbial life responds to microgravity, we may learn new ways to fight against them. There is little focus on the physical forces that could play a role in how pathogens cause disease. Everything we know evolved to live in gravity and once that constant force is removed, many interesting things happen and monitoring of the behavior is pertinent.
Along with bacteria, it has been found that lichens are extraordinarily adaptive to their surroundings. In 2005, two species of lichen–Rhizocarpon geographicum and Xanthoria elegans— were contained in a capsule and launched on a Russian Soyuz rocket. Upon reaching Earth’s orbit, the container opened, and the liches were exposed to the vacuum of space for 15 days before the lid resealed and returned to Earth. Along with the vacuum of space, they sustained life in various temperature (-20°C to 20°C) also to the ultraviolet radiation of the Sun. It was found that the lichens were in the shame shape as they were before being launched.15
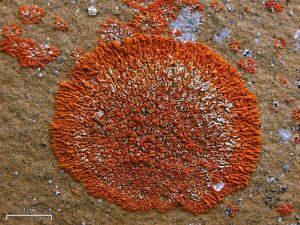
Figure 8– Xanathoria Elegans, one of the lichens mentioned, Credit: Jason Hollinger, Source: https://upload.wikimedia.org/wikipedia/commons/thumb/e/eb/Xanthoria_elegans_97571_wb1.jpg/1200px-Xanthoria_elegans_97571_wb1.jpg
Lichens protect themselves with a tough mineral coating that protects themselves from UV rays. On top of the coating, lichens are layered from individual organisms to individual organism which protects the underlying cells. It’s important what this might note for the implications this could provide in creating a farm on Mars without regards for Mars’ cold winters. This is what makes lichen remarkable creatures regarding their survival, however, the lack of oxygen in the vacuum of space would bring the eventual termination of the organism.15
Lichens are among many organisms that have shown great durable when exposed to various extreme environments that would otherwise be very harmful to complex life. These exceptional organisms are what we will focus on next, as the study and observations of these organisms could lead to further understandings of life can exist in space or not.
Extremophiles
Through these observations of microbial life, we are left questioning the versatility of microbial life. Complex life has a host of issues that make it problematic for survival in long periods in space shuttle environments, but as observed, microbes perform on a different level. Bacteria thrive in confined, warm spaces. However, outside of a space shuttle environment, can biology survive in space and harsh extraterrestrial climates? When we look for similar life to ours we search for signs of water, organic molecules, and sources of energy on planets, moons, and meteorites. However, signs of life have shown up in the most of extremely isolated and harsh environments. Here is a short video explaining extremophiles:
The video provided details the variety of harsh climates that certain microorganisms can withstand. Also introduced are the tardigrades or more colloquially known as “water bears”. These microscopic metabolic creatures create a form of stasis or cryptobiosis to survive a multitude of harsh circumstances.16 The study of tardigrades could lead into the right direction of how humans might be able to implement technology of interplanetary transportation through cryptobiosis. This is pertinent information as we proceed further into the age of space exploration.16

Figure 9– A tardigrade, the poster child for the many variations of extremophiles, Credit: Willow Gabriel, Goldstein Lab, Source: https://upload.wikimedia.org/wikipedia/commons/6/65/Hypsibiusdujardini.jpg
There have been many experiments testing the durability of extremophiles. One such ongoing project that is based in Carolos Chagas Filho Institute of Biophysics simulates inhospitable environments to test whether extremophile organisms can survive various situations. The organisms were exposed to intense radiation sources that simulated solar radiation equivalent to million years of accumulated dosage in the space of a few hours. The results revealed the extreme resistance of bacteria against many radiation sources (solar wind stimulants, X-rays, VUV) when protected by micro-sized carbonaceous grains.17
This suggests the possibility of microbial life elsewhere in the universe and the suggestion of transference of microorganisms via natural occurrence or panspermia.
Panspermia
Have you ever wondered where microbial life originated from? Panspermia is a theory that predicts microbial life could have been formed elsewhere and traveled between planets, inseminating units of life that could have been more complex in a habitable planet like ours. Panspermia is a Greek word that translates literally as “seeds everywhere”.1
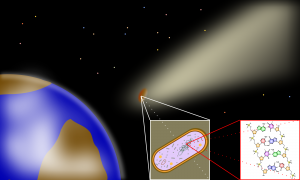
Figure 10– Depiction of comet transporting bacteria to Earth, Credit: Silver Spoon Sokpop, Source: https://upload.wikimedia.org/wikipedia/commons/thumb/4/48/Panspermie.svg/2000px-Panspermie.svg.png
The hypothetical endolithic microbial passengers would be forced through various situations such as acceleration, compression shock, and heating. Rock fragments are scattered upon the surface material of the destination planet, effectively releasing into the planet’s crust of water and potentially meeting the standards of hospitable environment conducive to growth.1
There are three variations of this theory: lithopanspermia, ballistic panspermia, and directed panspermia. Lithopanspermia postulates that impact-expelled rocks from a planet’s surface are transfer vehicles for spreading biological material from one solar system to another. Ballistic panspermia suggests the same situation as lithopanspermia, except that materials are exchanged within the same solar system. Directed panspermia is the theory of intentional spreading of life to other planets by an advanced extraterrestrial civilization, or human civilization spreading life to other planets.18
Theories such as panspermia provide a gateway of understanding towards microbial life and whether one supports the hypothesis or not, it does raise important questions considering our Last Universal Common Ancestor (LUCA). These theories allow us to narrow down our understandings and come closer to the truth about the origins of life in the universe.
Problematic Panspermia
There are a few issues when considering Panspermia as a valid theory. The main issue lies in the initial question, which asks how life emerged on Earth. However, an answer stating that life transcended from another planet is only evading the problem of where life originates. The answer does not explain how life emerged on the other planets and does not take into consideration what type of planets that these supposed organisms appeared from the beginning of the universe.1
Another issue arises if we find life like ours on another planet. The organisms we hypothetically detect could have been taken there from Earth, a sort of reverse panspermia. We cannot demonstrate that life on Earth came from where the organisms were detected making it very problematic to verify. Even further, this could create a crucial dilemma if we found living beings on Europa and could not decide on whether the beings were native extraterrestrial living beings of terrestrial living beings transported by our own space devices.1
More problems occur when considering the scientific method applied to the panspermia theory. When verifying a hypothesis, it demands the observation of natural phenomena. However, there have been no natural phenomena that could be connected to the panspermia hypothesis. This is what causes panspermists to have trouble performing experiments to verify their hypothesis. For it to be verified, physical evidence must be applied and tested thoroughly.1
Given that evolution on Earth seems to have passed through protocellular evolutionary stages of progenotes, this would appear to be incompatible with the panspermia theory because this observation would imply that the infection bringing life to the Earth started in these protocells, for which a low or null infective power is generally expected.
In addition to all these refutations, there is far more valid evidence supporting the theory of life originating on Earth. This video explains how biological evidence supports the theory of life beginning on Earth:
Conclusion
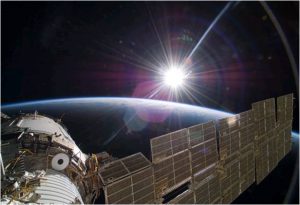
Figure 11– The bright sun greets the International Space Station from the Russian section of the orbiting laboratory, Credit: NASA, Source: https://blogs.nasa.gov/wp-content/uploads/sites/207/2013/06/1027358main_ISS_sunrise.jpg
In conclusion, we’ve come to understand that microbial life is far more robust than complex life, as various microbes demonstrate the ability to adapt to usually detrimental environments i.e. radiation, high temperatures, low temperatures, vacuum of space, etc. It’s also extremely important in maintaining the cleanliness on board a space shuttle, otherwise the detrimental effects of microbial life could completely ruin a space mission if not closely monitored. But, one can never be too certain about the outcome of microbial life, as microgravity has an undetermined effect on the virulence of bacteria. Lichens have evolved to be fortified with their layers of cells making it able to survive the vacuum of space and bacteria behave extraordinarily different in a microgravity environment as observed by various space agencies.
We are left considering the question of whether life can exist in space. As displayed by the observations made from extremophiles, yes, life can certainly live in the vacuum of space. Tardigrades create their own cryptobiosis state, bacteria develop “shields” to protect themselves from radiation, and lichens have layers of cells protecting the core of their livelihood. However, many of these extremophile types are unfortunately very understudied and need further analysis to determine the full measure of their extreme behavior.
We’ve also touched upon the theory of Panspermia and the issues that arise considering its hypothesis and avoidance of answering the question of where life originates from. There are still many people claiming that this hypothesis still holds merit as it attempts to solve one of life’s greatest mysteries considering its origin.
What does this mean for mankind? We’ve also discovered more immediate concerns toward the well-being of humans. Astronauts have determined faster ways to analyse the presence of bacterial life using the LOCAD-PTS, and are further developing more advanced technologies to diagnose illnesses using saliva or blood samples. Along with the study on the effects of radiation-resistant microbes could further the understanding of how infections work, the more we see how microbes function in different settings, the more we can understand it.
If you care to disregard the importance of microbial life, remember this: what you can’t see can’t hurt you, until microbes begin eating away at the components of your space shuttle and form brown basketball sized globules.
Works Cited
1 N. Nasif, (2000), Panspermia, WWW Document, http://sites.usask.ca/astr104/t201609/how-does-position-affect-geology/
2 S. Castro, D. Smith, C. Ott, (2014), Microbial Observatory Mini Book: NASA ISS Program Science Office, PDF Document, https://www.nasa.gov/sites/default/files/files/Microbial-Observatory-Mini-Book-04-28-14-508.pdf
3 G. Hubbard, Astrobiology: Its Origins and Development, WWW Document, https://www.nasa.gov/50th/50th_magazine/astrobiology.html
4 T. Bell., (2007), Preventing “Sick” Spaceships, WWW Document https://science.nasa.gov/science-news/science-at-nasa/2007/11may_locad3
5 T. Ichigo, N. Yamaguchi, F. Tanigaki, M. Shirakawa, M. Nasu, (2016), Four-year bacterial monitoring in the International Space Station-Japanese Experiment Module Kibo with culture-independent approach, npj Microgravity 2, Article number: 16007, Retrieved from https://www.nature.com/articles/npjmgrav20167
6 M. Ott, D. Pierson, M. Shirakawa, F. Tanigaki, M. Hida, T. Yamazaki, T. Shimazu, N. Ishioka, (2014), Space Habitation and Microbiology: Status and Roadmap of Space Agencies, Microbes Environ., 29(3): 239–242, Retrieved from https://www-ncbi-nlm-nih-gov.cyber.usask.ca/pmc/articles/PMC4159034/
7 NASA, (2010) What is Microgravity?, WWW Document, https://www.nasa.gov/audience/forstudents/k-4/stories/nasa-knows/what-is-microgravity-k4.html
8 B. Christensen, (2007), Unwanted Life Forms Abound in Sick Spacecraft, WWW Document, https://www.space.com/3832-unwanted-life-forms-abound-sick-spacecraft.html
9 J. Rosenzweig, W. Abogunde, K. Thomas, A. Lawal, Y. Ngyuyen, A. Sodipe, O. Jejelowo, Spacecraft and modeled microgravity effects on microbial growth and virulence, Applied Microbiology and Biotechnology, Volume 85, Issue 4, pp 885–891, Retrieved from https://link-springer-com.cyber.usask.ca/article/10.1007%2Fs00253-009-2237-8
10 L. Pourcelot, F. Patat, M. Defontaine, J. Gregoire, M. Berson, Microgravity, Ultrasound in Medicine and Biology, Volume 26, Supplement 1, May 2000, Pages S144-S146, Retrieved from http://www.sciencedirect.com.cyber.usask.ca/science/article/pii/S0301562900001903?via%3Dihub
11 NASA, Lab-on-a-Chip Applicational Development-Portable Test System (LOCAD-PTS), WWW Document, https://www.nasa.gov/mission_pages/station/research/experiments/232.html
12 Love. S, (2016), Bacteria get dangerously weird in space, WWW Document, https://www.washingtonpost.com/news/to-your-health/wp/2016/10/25/bacteria-get-dangerously-weird-in-space/?utm_term=.6408acbb8039
13 S. Stirone, (2017), The Quest to Kill the Superbug That Can Survive in Outer Space, WWW Document, https://www.theatlantic.com/science/archive/2017/03/bacteria-in-space/520908/
14 M. Knight, (2017), Space spores: NASA’s struggle with radiation-resistant microbe could help us understand infections, WWW Document, https://geneticliteracyproject.org/2017/05/25/space-spores-nasas-struggle-radiation-resistant-microbe-help-us-understand-infections/
15 K. Young, (2005), Hardy lichen shown to survive in space, WWW Document, https://www.newscientist.com/article/dn8297-hardy-lichen-shown-to-survive-in-space/
16 R. Courtland, (2008), ‘Water bears’ are first animal to survive space vacuum, WWW Document, https://www.newscientist.com/article/dn14690-water-bears-are-first-animal-to-survive-space-vacuum/
17 C. Lage, G. Dalmaso, L. Teixeira, A. Bendia, I. Paulino-Lima, D. Galante, E. Janot-Pacheco, X. Abrevaya, A. Azua-Bustos, V. Pelizzari, A. Rosado, (2012), Mini-review: Probing the limits of extremophilic life in extraterrestrial environment-simulated experiments, PDF Document, https://arxiv.org/ftp/arxiv/papers/1207/1207.2098.pdf
18 (2017), Panspermia Theory, WWW Document, http://www.panspermia-theory.com/