Exploring the Far Side of the Moon
Hanna Dick, Daniel Glisic, Brooklyn Miller, and Alice Ok
For billions of years, light from the sun reflected from the near side of the Moon, matching its orbit with Earth’s rotation. This offered the well-recognized image of the Moon that resonates with our mind since we first looked towards the night sky. This also left the far side of the Moon a mystery. Interest towards this mystery peaked during the Space Race, when Luna 3 in 1959 captured photos of the lunar far side, and the Apollo missions (starting with Apollo 8) offered astronauts the opportunity to view it directly.12,14 Observations since then continued this trend of looking from afar, using various telescopes on orbiters to capture this data, and spectrometers to gather information about the lunar far side’s chemical distributions. It was not until China’s Chang’e-4 mission launched in December of 2018 that data could be gathered directly from the Moon’s far side surface. We will observe some background information, some of the mechanics behind the Chang’e-4 mission, and touch on the value of the data collected, to get an understanding of the process behind studying the far side of the Moon.

Figure 1 – Illuminated ‘dark side’ of the moon NASA’s Earth Polychromatic Imaging Camera (EPIC) Source: https://www.nasa.gov/feature/goddard/from-a-million-miles-away-nasa-camera-shows-moon-crossing-face-of-earth
Why Can’t We See the Far Side of the Moon?
The far side of the Moon remains invisible to us, due to tidal locking; a process where a satellite’s orbital motion is synced with the celestial object’s rotation.13 This leads to the near side of the Moon always facing Earth, seen in Figure 1 and 2, and the far side of the Moon always covered and seemingly impossible to see from our perspective. Observations from Earth only created deductions based on the near side of the Moon, as there was no way to gather evidence of what the other side was like, let alone how it would differ from others.

Figure 2 – Tidal Locking of the Moon with the Earth Source: https://commons.wikimedia.org/wiki/File:Tidal_locking_of_the_Moon_with_the_Earth.gif
Evidently, they learned about parts of the lunar far side later through understanding a process called libration.19 It is when the Moon appears to slowly wobble while facing the Earth, allowing for 18% of the far side to be visible over the period of a month due to the change in Earth’s perspective.19 The angle the far side appeared from Earth was steep, which made mapping and observations difficult.19 More importantly, 82% of the far side remained hidden away.19
This left astronomers feeling curious, wanting to explore. Wondering what was really on the far side of the Moon. The desire to learn about the remaining 82% led to astronomers using orbiters such as the Lunar Reconnaissance Orbiter (LRO), seen in Figure 3, to map out the lunar far side.15

Figure 3 – Lunar Reconnaissance Orbiter (Artist’s Concept) Source:https://www.jpl.nasa.gov/spaceimages/details.php?id=PIA18163
The LRO utilizes instruments such as the Lunar Reconnaissance Orbiter Camera and the Lunar Orbiter Laser Altimeter for creating a 3D high-resolution image of the far side of the Moon and recording the illumination of each of the areas to understand its depth respectively.15 Using this information, astronomers named the visible craters, maria, and other phenomena on the lunar far side.
They utilize this information to observe the relative locations of craters and pay homage to significant individuals, such as naming a crater after Enrico Fermi, known for creating the first controlled nuclear reaction, and the Fermi paradox.10
Using this information, astronomers named the visible craters, maria, and other phenomena on the lunar far side. They utilize this information to observe the relative locations of craters and pay homage to significant individuals, such as naming a crater after Enrico Fermi, known for creating the first controlled nuclear reaction, and the Fermi paradox.10 Alongside this information, they utilized information from various spectrometers within the orbiter and compared it to known elements, to determine information about the far side’s composition, and distribution of those minerals.15 It is through prior observation and interpretation, that has led us to know what we know about the lunar far side. However, there still remained a desire to push that boundary and one day land on the far side of the Moon. That desire came true when Chang’e-4 launched to land on the far side of the moon in January 2019.
Chang’e 4 Mission
The Chinese National Space Administration (CNSA) formed the Chinese Lunar Exploration Program with the goal of observing the distribution of energy, minerals, and lunar environments, learning about ways to utilize it, and finding the best place for a lunar base.26 This program was organized in three phases, with each phase made up of robotic lunar missions.26 For the first phase, they sent orbiters to the Moon to map it out in detail, similar to the Lunar Reconnaissance Orbiter.26 The Chang’e-4 is part of the second phase, where they deploy rovers to observe the lunar surface.26 For the third phase, they plan to collect lunar material (such as rocks or minerals) and return it to Earth.26 These missions have secondary goals of exploring the lunar surface, and observing the far side’s topography, composition and subsurface structure, based on information from the landing area.26 All of this is meant to lead to an eventual manned mission to the Moon.21 The program has also received support from other countries, including Germany and Sweden.22 The international support extends the abilities of the CLEP, allowing for more experiments to be done, and payloads to be used during mission.22 We then come to now, as we come to understand how each of the payloads in the Chang’e-4 mission play a larger role in understanding the world around us.
Payloads
Lunar Lander
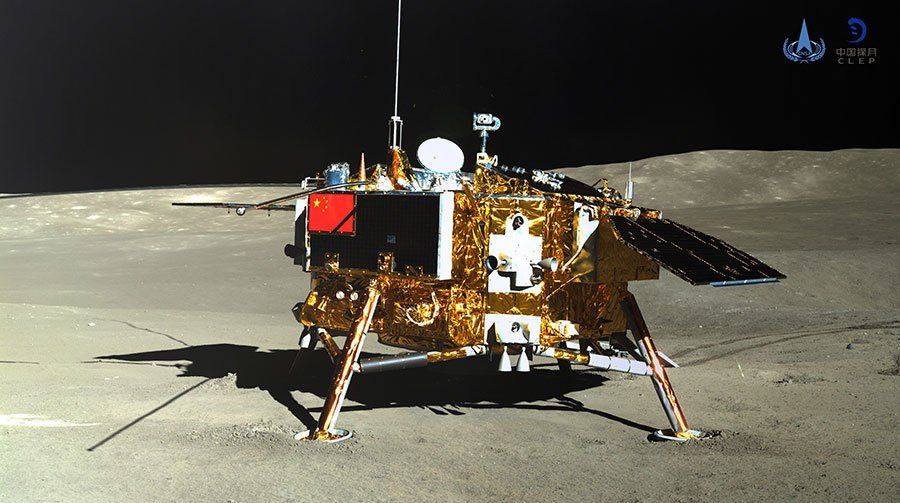
Chang’e 4 Lunar Lander taken by Yutu 2 Source: https://twitter.com/CHANG_E_4/status/1084338571840876544
The first payload of the Chang’e 4 lander is to provide power to each of the payloads, using radioactive isotopes designed to handle hibernation during the lunar nights.22 The second is creating a cache to allow for data to buffer during preprocessing.22 The last is to provide commands for operating all of the payloads, to allow them to function properly.22 On top of the control over payloads, it also utilizes itself to process, control, and store data gather data from payloads, to be transmitted by satellite.22 Through this, the lander remains a hub for providing support for the payloads and information for the Chang’e-4 mission.
The landing camera (LCAM) is mounted on the bottom of the Chang’e-4 lander, pointed towards the ground for observation.22 It continually takes video during its descent into the lunar surface starting at around 12 km (recording 1,656.9 m at a 2 km elevation to 3.3 m at a 4 m elevation), until it touches the ground.5,22 It collects information through a charged-coupled device (CCD) imaging sensor, which converts light waves into electrons through hitting one or more of its cells.8 CCD is used due to producing less visible noise as a result of movement during landing.8 This forms electrons within the sensor that travel to one of the corners of the array to record the electrons as digital values understandable by a computer.8 Following this, the data transfers to the signal processing board used to control the main payload based on instructions from the lander, and provides power to the capturing assembly itself.22 The board also controls for exposure, compresses the image, and prepares the image for data transmission to the lander for storage and transmission to the satellite.22 Eventually, data gets transferred through the low-voltage differential signalling (LVDS) driver.20,22 This process can be viewed in the following link: https://ibb.co/b7Yth8t22
In a simplified explanation, this kind of signalling involves two components, the host transmits binary data over several conductors to the target controller, allowing for multiple bits of data to be transported at once.20 Through this, we can store 1024×1024 images and video footage of the Chang’e-4’s landing, allowing us to map the landing site as we land, ensuring the landing goes smoothly.22 It can also offer us potential on learning more about the landing site, and how viable it may be for future missions.
The Terrain Camera creates panoramic photos by taking a photo every 19 degrees, or 19 images for each circle, allowing for imaging within a 600-meter radius.5 It also utilizes colour complementary metal-oxide-semiconductor (CMOS) cameras which are located on a bar that is able to rotate 360 degrees on top of the lander and move 120 degrees up or down for a panoramic view of the lunar surface.22 The composition diagram of the terrain camera can be viewed through the following: https://ibb.co/d6JDQT922
They use CMOS imaging sensors to record the images (to save costs and power as the lander is still) using transistors for each pixel and traditional wires to transfer the charge.8,22 The imaging data then goes through a dedicated image compression module, while being managed by the RS422 interface using commands from the lander.22 The imaging data is then transmitted back to the lander for storage and transmission.22 Data from the terrain camera can offer an understanding of the distribution of the geographical terrain near the landing area, or for viewing the Moon surface for minerals and soils. The terrain camera can also allow engineers to observe the rover’s functionality, and the functioning of each of the payloads as it conducts scientific investigation, to ensure that the rover and its payloads are working properly.22
The new low-frequency spectrometer uses a tricomponent active antenna element sticking partially outside the lander cabin to explore the Moon’s ionosphere.22 A spectrometer analyzes a band of light separated similarly to a prism, and this data can be used for referencing the emission spectrum to the emission spectrum of known elements to determine the contents of the atmosphere.25 The reason for using three antennae instead of one is because it can capture information about the intensity, spectrum, time variation, polarization, and direction of the radiation through analysis of three component data not possible to gather by only one antenna.22 The three receiving antennae collect the data in a spectrum range from 420-700 nm, go through a preamplifier, and then inside the lander cabin to the electrical unit.22 The electrical unit contains the interface control that transfers the information to a multi-channel receiver, and later to the controller.22 The controller is used to obtain and direct instructions to and from the lander, and spread the instructions to the other parts of the payload.22 The data collected would be taken to an interface, to be eventually transferred to the data bus for storage in the lander.22 Through this process, we can observe the ionospheres of space, without any interference from electromagnetic radiation coming from Earth. The ionosphere would be important when observing how radio waves would be affected when entering the lunar atmosphere.22 The composition of the Low-Frequency radio spectrometer system can be viewed through the following: https://ibb.co/ZRr9C5922
This could provide for an opportunity to know whether to add radio telescopes on the far side’s lunar surface, as they would be able to gather electromagnetic radiation without interference from the ionosphere or noise from Earth.
Also notable on the lander was an attempt at maintaining an ecosystem in the Yutu-2 by Chongqing University.6 Using two tanks, they would send one to the far side of the Moon and leave another at the university to comparing the growth and development of an ecosystem.6 This was to see if there are any differences in development due to differences between the Moon and Earth.6 To form the ecosystem, the seeds of six organisms were placed in the tank (Figure 4), containing four producers (potato, rape seed, cotton, arabidopsis) a consumer (fruit fly), and a decomposer (yeast).6
Lunar Rover Yutu 2
The rover itself has similar abilities to the lander, especially how it contributes to payloads.22
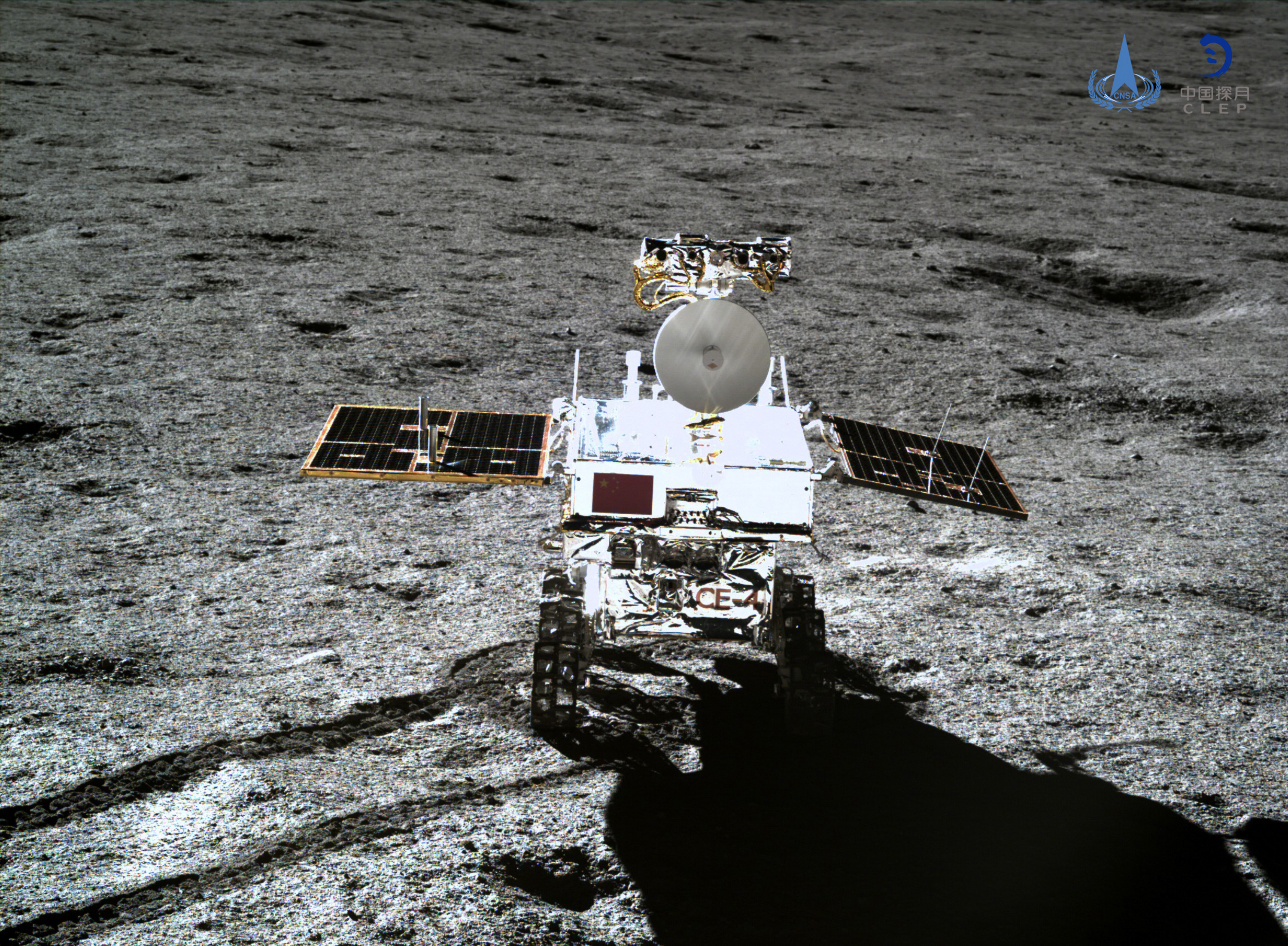
Chang’e 4 Yutu 2 taken from Landing Terrain Camera Source: https://twitter.com/CHANG_E_4/status/1084339378862706688
What makes it unique is its ability to move around the lunar surface.22 It uses six wheels and a rocker-bogie suspension to move around.9 The suspension works similar to the Curiosity Rover, by allowing the wheels to swivel around the track, while the differential keeps the rover body balanced regardless of the position of the wheels.16 When one side goes up, the other side goes down to counteract the balance.16 All of the payloads and their movement are powered by sunlight, thanks to two wide solar panels fitted into the rover.9 Payloads are either placed on the rover’s main body, or placed on its robotic arm.9 The rover itself provides similar functioning to the lander, powering each of the payloads, creating a cache for data to buffer, providing commands, and can even transmit information through to the satellite to ground control.22 They also promote communication between the rover and the lander, either allowing the lander to be used for storage or transmitting other commands towards the rover.22
Unlike the terrain camera, the panoramic camera mounted on the Chang’e 4 rover is made up of one large electrical unit connecting the optical system, the mechanical system, the electronics and thermal control units all together.22 The panoramic camera is made up of two CMOS sensors installed on the lunar rover mast. able to rotate a full 360 degrees to get a 3D panoramic view.22 The composition of the panoramic camera can be viewed through the following: https://ibb.co/qk9mmtG22
The CMOS sensors also collect the data and send image date through a series of sequences involving exposure, camera control, image, caching and other forms shown in figure #. These sequences also interact and send data which affects the capturing of wavelengths through the CMOS detector.22 There also is a time module powered by a crystal oscillator for keeping track of time.22 From there, data gets transferred through LVDS (Low Voltage Differential Signaling), and reaches the rover.20,22 Thus, this creates a visual of the lunar surface without being constrained in one spot.22 This allows us to map out the Moon’s surface further than what the satellite would be able to see, and it can allow us to explore the far side of the Moon for the first time.
The lunar penetrating radar (LPR) uses its nanosecond impulse radar to detect the structure of the lunar subsurface and lunar regolith (i.e. the solid structure above bedrock), calculating its thickness and its structure.22 This is based on the principle that some radio waves would pass through the different layers of the Moon, while reflecting off of others.7 The two transmitting antennae creates an impulse of radio waves within a time frame, orchestrated by the controller and individual transmitters, that reflects from the lunar surface back to the antennae, amplified by the receiver, and then recorded.22 From there, it goes through the controller, where information is transferred to the lander’s data bus.22 This allows for not only learning about the morphological terrain of the lunar ground, but can also provide for evidence to support the study of the formation and evolution of the Moon.7 We could use this to understand if there may be anything underneath as well, such as rare metals or seeing the formations of valuable resources. The composition of the LPR system can be viewed through the following: https://ibb.co/QJv44pr22
The infrared imaging spectrometer (or VNIS which stands for visible near-infrared spectrometer) utilizes a sensor and electronic units to view the near-infrared and visible spectrum of objects on the lunar surface.22 The purpose of this is to provide information towards determining the lunar surface’s mineral composition, including pyroxene, plagioclase, olivine, ilmenite, and possibly new and undiscovered minerals.27 It calculates the composition of things in the near-infrared and visible light spectrums.22 They are separated into two parts, the detector electric unit, and the spectrum control electric unit.22 The detector electric unit, passing through a calibration module (that also provides dust protection), which captures the visible information through a series of modules (imaging forming lens, aperture, ion lens, etc) meant to get the proper conditions to capture the emission spectrum onto two visible detectors that detect the information and allows for data to be collected.22 Acousto-optic tunable filters (AOTFs) that allow for determining different wavelengths over the available spectral coverage. ranging from 450 to 2400 micrometers.27 The spectrum control electric unit offers secondary power, control over what part of the spectrum they are looking for, and processing and eventually transferring the data.22 These two units collaborate to offer data about the lunar surface’s composition, allowing us out of pure curiosity about the Moon, and to determine the Moon’s resources, for a possible future Moon base. The system design of the VNIS can be viewed through the following: https://ibb.co/RNFt6Nw22
Lastly, the Advanced Small Analyzer for Neutrals studies the memory mechanism of the lunar surface’s sputtering process in the lunar atmosphere, and the energy spectra of energetic neutral atoms from reflected solar wind ions under different solar wind illumination conditions.11,22 Sputtering is when particles are ejected from a solid target material through bombardment from energy particles.22 These energy particles come from the sun, like protons, electrons, and atoms are ejected at a velocity of anywhere between 20-2000 km/sec, either being deflected from the magnetic fields of a planet or hitting its surface. The Advanced Small Analyzer is simply a small energetic neutral particle sensor.22 This was created with support from the Institute of space physics in Sweden as a part of an international collaboration.22 The point of bringing this analyzer is to determine how the near-surface environment looks, and why the scattering rate from solar wind interaction so high on the Moon.11 This allows us to determine the risk we may experience from the solar radiation, and whether we should look further into actions to protect ourselves from these energy particles should a manned mission to the Moon happen.
Satellite Queqiao
Without the satellite, we would be unable to gather any information from the far side, due to being blocked off physically by the Moon from the Earth, or too far away to maintain an orbit with the Earth and the Moon.17 Queqiao relays information between the lander, rover, and the ground station, while orbiting around a unique orbit called L2.17 Using microwaves, the satellite relays from ground stations through TT & C (telemetry, tracking, and command) and relays from the lander and rover through BPSK (binary phase-shift keying).17 TT&C is an abbreviation of signals used for transmitting the health and status updates for the satellite, locating and following the satellite, and controlling the satellite.23 BPSK represents a way of sending binary information using two different phase states to represent 0 and 1, allowing for efficient transmission of data from the rover and lander back to Earth.17,24 The advantage of this is its simplicity, as few all it needs to know if the relative position of the wave on an axis, being either 0 degrees for 0, or 180 degrees for 1.24 Through this process, as seen in Figure 6, data from the lander and the rover can effectively be transferred to Earth for research.
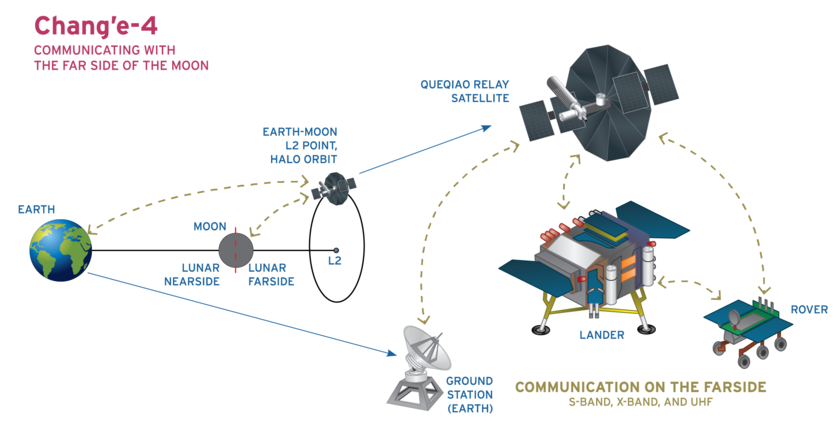
Figure 6 – Communicating with the far side of the moon Source: https://twitter.com/CHANG_E_4/status/1084339378862706688
With support from Radboud University Nijmegen in the Netherlands, the satellite on the Chang’e 4 mission features one payload, the Low-Frequency Explorer.22 This payload explores the far side of the Moon’s promising potential to search for low-frequency radio signals, without interference from Earth’s ionosphere.22 The Low-Frequency Explorer falls into the category from 1 – 80 MHz and is particularly difficult to detect without putting it out into space, as these frequencies cannot be emitted on the Earth’s ground and are interfered by auroral kilometric radiation noise and other manmade radio frequencies.22 However, the far side of the Moon provides a stable environment in which the low-frequency radio will be sheltered from these disturbances.22 The satellite payload will conduct a survey of our Galaxy and will help create a resolution map of the radio sky with this information. The satellite will also explore some of Earth’s auroral kilometric radiation noise and possibly some of the planetary radio bursts from places like Jupiter.22 What we might learn from these observations will hopefully bring more light to space weather events, or perhaps assist us in searching for extraterrestrial life within our galaxy.22
What does this mean for the future?
The Chang’e-4 represents the next chapter in understanding the far side. This represents a unique moment where we can interact with the lunar surface, experiment on ways to sustain life on the Moon, and work towards planning a future lunar base. There is value to colonizing the Moon, whether to have a place to sustain life, to serve as an intersection for interstellar travel, and/or as a place to gather more resources, and as evidence comes in, plans to travel there will be more probable and realistic. Information from the mission continues to be gathered, yet as the mission is gathering and analyzing data, little has arisen. The success of the maintenance of the rover through the cold nights on the Moon and the sprouting and subsequent death of the plants within the contained ecosystem are the only two examples found so far.1,2 While this doesn’t teach us about the far side of the moon, it teaches us what works, and more importantly, what ends up not working, and how we can prevent it from happening the next time they land a rover on the Moon. Over towards the Chinese National Space Agency, the Chang’e-4 is considered a complete success, gaining distinction through a visit from Xi Jinping for their actions.3,4 The support and excitement from the Chang’e-4 could encourage their space program to continue, and lead other countries to pursue space travel on their own time. It just shows that sometimes you need to take a new angle, and take a great leap, before others will take interest and follow.
Sources
1 A. Jones. Lunar nighttime brings end to Chang’e-4 biosphere experiment and cotton sprouts. https://gbtimes.com/lunar-nighttime-brings-end-to-change-4-biosphere-experiment-and-cotton-sprouts. (2019)
2 CNSA. China’s Chang’e-4 probe resumes to work after lunar night. http://www.cnsa.gov.cn/english/n6465652/n6465653/c6805358/content.htm. (2019)
3 CNSA. China declares Chang’e-4 mission complete success. http://www.cnsa.gov.cn/english/n6465652/n6465653/c6805233/content.html. (2019)
4 CNSA. Xi meets Chang’e-4 mission representatives. http://www.cnsa.gov.cn/english/n6465652/n6465653/c6805507/content.html. (2019)
5 C. Li · J. Liu · X. Ren, W. Zuo, X. Tan, W. Wen, H. Li, L. Mu, Yan Su, H. Zhang, J. Yan and Z. Ouyang. The Chang’e-3 lander and rover mission to the Moon. https://link.springer.com/article/10.1007/s11214-014-0134-7. (2014)
6 Chongqing University. Change-4 Probe lands on the moon with “mysterious passenger” of CQU. http://english.cqu.edu.cn/info/1038/1941.htm (2019)
7 G. Fang, B. Zhou, Y. Ji, Q. Zhang, S. Shen, Y. Li, H. Guan, C. Tang, Y. Gao, and W. Lu. Lunar Penetrating Radar onboard the Chang’e-3 mission. https://iopscience.iop.org/article/10.1088/1674-4527/14/12/009/meta. (2014)
8 K. Nice, T. V. Wilson, and G. Gurevich. How Digital Cameras Work. https://electronics.howstuffworks.com/cameras-photography/digital/digital-camera2.htm (2006)
9 K. Tate. How China’s Chang’e-3 Moon Rover Yutu Works (Infographic). https://www.space.com/23855-how-china-change3-moon-rover-works-infographic.html. (2013)
10 L. Badash, Enrico Fermi. https://www.britannica.com/biography/Enrico-Fermi. (2019)
11 M. Wieser, S. Barabash, A. Grigoriev, and Z. Aibing. The Advanced Small Analyzer for Neutrals (ASAN). http://impact.colorado.edu/dap/abstracts/martin_wieser.pdf. (2017)
12 NASA. Apollo 8. https://www.nasa.gov/mission_pages/apollo/missions/apollo8.html (2009)
13 NASA. Earth’s Moon. https://solarsystem.nasa.gov/moons/earths-moon/overview/ (2019)
14 NASA. Luna 3. https://nssdc.gsfc.nasa.gov/nmc/spacecraft/display.action?id=1959-008A (2018)
15 NASA. Lunar Reconnaissance Orbiter (LRO): Leading NASA’s Way Back to the Moon. https://www.nasa.gov/pdf/360020main_LRO_LCROSS_presskit2.pdf. (2009)
16 NASA. Wheels and Legs. https://mars.nasa.gov/msl/mission/rover/wheelslegs/. (2008)
17 P. Ye, Z. Sun, H. Zhang, and F. Li. An overview of the mission and technical characteristics of Change’4 Lunar Probe. http://engine.scichina.com/publisher/scp/journal/SCTS/60/5/10.1007/s11431-016-9034-6?slug=fulltext. (2017)
18 R. F. Wimmer-Schweingruber, S. Zhang, C. E. Hellweg, Jia Yu, J. Guo, H. Lohf, T. Berger, S. I. Böttcher, S. Burmeister, A. Knappmann, V. Knierim, B. Schuster, L. Seimetz, H. Woyciechowski, G. Shen, and B. Yuan, The Lunar Lander Neutron & Dosimetry (LND) Experiment on Chang’E-4. https://www.hou.usra.edu/meetings/lpsc2017/eposter/1320.pdf. (2017)
19 P. D. Spudis, Moon. https://web.archive.org/web/20130703162844/http:/www.nasa.gov/worldbook/moon_worldbook.html (2004)
20 Texas Instruments. Low Voltage Differential Signaling (LVDS). http://www.ti.com/lit/an/slla014a/slla014a.pdf. (2000)
21 T. Nowakowski. China eyes manned lunar landing by 2036. https://www.spaceflightinsider.com/organizations/china-national-space-administration/china-eyes-manned-lunar-landing-2036/. (2017)
22 Y. Jia, Y. Zou, J. Ping, C. Xue, J.Yan, and Y. Ning, The scientific objectives and payloads of Chang’E−4 mission, https://www.sciencedirect.com/science/article/pii/S0032063317300211 (2018)
23 J. E. Keesee. Satellite Telemetry, Tracking and Control Subsystems. https://ocw.mit.edu/courses/aeronautics-and-astronautics/16-851-satellite-engineering-fall-2003/lecture-notes/l20_satellitettc.pdf. (2003)
24 M. Viswanathan. Binary Phase Shift Keying (BPSK) – modulation and Demodulation. https://www.gaussianwaves.com/2010/04/bpsk-modulation-and-demodulation-2/. (2010)
25 W. Harris. How Laser Analysis Works. https://science.howstuffworks.com/laser-analysis2.htm. (2008)
26 Z. Ouyang. The Scientific Objectives of Chinese Lunar Exploration Project. https://web.archive.org/web/20071026112423/http://www.geog.umd.edu/ispmsrs2005/OuyangZiyuanAbs.htm (2005)
27 Z. P. He, B. Y. Wang, G. Lv, C. L. Li, L. Y. Yuan, R. Xu, K. Chen, and J. Y. Wang. Visible and near-infrared imaging spectrometer and its preliminary results from the Chang’e-3 project. https://aip.scitation.org/doi/pdf/10.1063/1.4891865. (2014)