By Foster Herriot, Jack Huang, Callan Kimber, Jadyn Kimber, Rob Newton, Hannah Roberts and Louisa Selby
Modern technology has allowed for humans to see further into the universe than ever before. Since the time of the space race, space missions have been used to conduct direct exploration of the universe, providing us with information and insight that earth-based observation alone cannot obtain. Many recent discoveries have been made through unmanned space missions, including the very recent and crucial discovery that there is liquid water on Mars.
Mars is one of the most important areas for space exploration today. As one of our neighbouring planets, it is likely to be the next step explorers take in our venture to the stars. As far as we know, a planet’s viability for the existence of life depends on the presence of water, so the search for water in the universe is ongoing. We know this through data gathered by missions dating back to 1971. This report will examine the historical context of this discovery, the technology and methodology involved, and the implications for future space exploration. It will help us truly appreciate the significance of their findings.
History
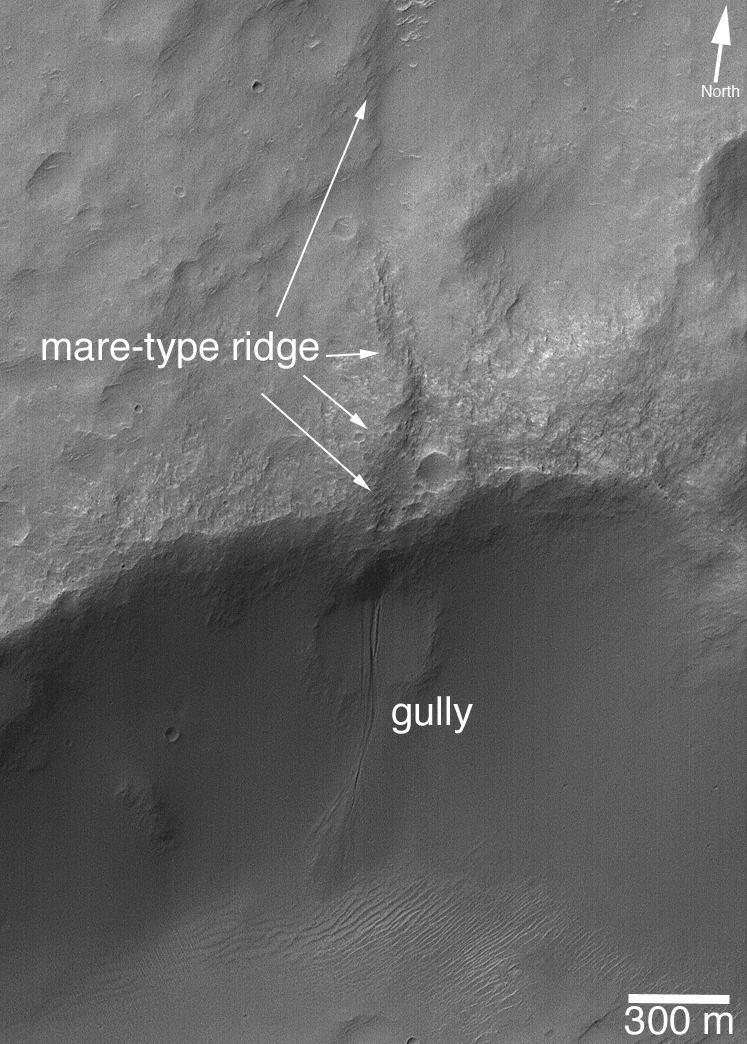
Image of Martian features likely caused by liquid water, taken by the MGS.5
Courtesy of NASA/JPL/Malin Space Science Systems.
The search for water in the universe and the exploration of Mars have often gone hand in hand, due to the proximity of the planet. Images taken by Mariner 9 as early as 1971 provided the first empirical evidence that water once existed on Mars—pictures of erosion on canyons, as well as dry riverbeds.1 The Viking Rover program of 1976 found a correlation between the existence of magnesium sulfates on the planet and the possibility that there was liquid water on ancient Mars.1
The Mars Global Surveyor (MGS) was the first successful US mission to Mars since the Viking missions. It arrived on September 11, 1997, and its purpose was to gain a better understanding of Mars’ seasonal changes. The mission discovered the migration of water vapor from hemisphere to hemisphere and gave scientists their first three-dimensional view of Mars’ topography. Using these findings, along with a high-resolution camera and a mineral detector, scientists were able to find gullies. They also discovered hematite, a mineral formed in water. These are both indications of the existence of water in the past. Regions of gullies and debris flow suggested that there could still be liquid water near the surface of Mars, and this exciting new evidence ignited a revival of Mars exploration all over the world.2
In December 2000, photographs from Mars revealed that sedimentary rock layers had filled impact craters near the equator of Mars, and water was likely responsible for the build-up of those rocks.3 For the decade following 2001, the US launched spacecraft to Mars at every opportunity, about every 26 months. The mission’s original strategy included using data from international missions, as the exploration for water and life on Mars is a world-wide initiative.4
Mars Odyssey launched April 7, 2001 and arrived in Mars’ orbit on October 23, 2001. Its primary goal was to map Mars in order to analyze the chemical and mineralogical composition of its surface, while also analyzing infrared wavelengths. In 2008, it located over 200 separate places on the south side of Mars that contained salt. It is theorized that water existed in these locations in the past. However, due to the fact that the sites are not physically attached, they are unlikely to be part of an old ocean. In 2010, the Odyssey reached its goal of mapping Mars, with 21,000 THEMIS images.5 In 2004, two of NASA’s rovers, Opportunity and Spirit, launched and also found evidence of water in Mars’ past.1 In 2007, NASA launched the Mars Phoenix, a stationary lander, and it found water ice beneath the planet’s surface.1
The Mars Curiosity Rover launched in 2013. It found areas on Mars that were previously covered by water, in addition to organic compounds that can be considered the fundamental building blocks of life as we know it.1 Landforms suggest the location of past bodies of water—the flat plains of the northern hemisphere are believed to be the former location of ancient oceans, while rivers and gullies suggest where water once flowed. A channel system called Marte Vallis may have had more than 100 times as much water flowing in it annually than the Mississippi River. The Mars Curiosity Rover discovered that at least one region of Mars, Mount Shap, was built by sediments, which suggested that large pools would have existed for an extended period of time.1 It is predicted that some of Mars’ water is now at the polar caps, while more is underground.6
The Mars Reconnaissance Orbiter
The most recent discovery that there is currently liquid water on Mars was achieved by the Mars Reconnaissance Orbiter (MRO). The MRO is a multipurpose spacecraft that weighs less than 2100 kg. It was launched from Earth on August 12, 2005 and arrived on Mars on March 10, 2006. It used an aerobrake system and the Martian atmosphere to slow it down, and has been orbiting Mars ever since.8
The following video shows some of the magnificent views captured by MRO during its first decade orbiting Mars.
MRO Technology
The MRO utilizes many technological instruments for conducting its research. It uses high-tech KA band radio telecommunications equipment allowing for efficient and complex communication with Earth, not only from itself but from other Mars-based spacecrafts.8 This communication system is like an “interplanetary internet” that will be used by many missions in the future.8
The CRISM (Compact Reconnaissance Imaging Spectrometer) is the only spectrometer aboard the MRO, but there are many other instruments mounted on it for Martian observation.
For cameras and imaging devices, it has the HiRISE (High Resolution Imaging Science Experiment), a large and powerful camera used for capturing visible light and producing powerfully enhanced images. It has the CTX (context camera), a wide-angle camera for producing less zoomed images that give geographical context to the more zoomed in ones. To keep track of the Martian climate, it has the MARCI (Mars Color Imager), that takes visible light and ultraviolet photographs charting the Martian climate’s temperature, and keeps track of weather features like ozone, dust, and C02 quantities in the atmosphere. A prototype of an experimental navigation camera, sort of like an eye, may in the future be used to help guide the landing of rovers and probes visually.8
The MRO uses the MCS (Mars Climate Sounder), which takes photographs of the Martian horizon in 9 different channels that detail the different wavelengths of light it can observe. Doing this, it can create a detailed 3D map of the Martian atmosphere and changes in its climate and composition. Lastly, it has the SHARAD (Shallow Radar), a device that produces radio waves, which penetrate shallowly into the Martian surface. The return signals help researchers determine the presence of water below the surface of Mars.
Spectrometers
Spectrometer technology played a major role in the discovery of liquid water. Spectrometers are devices that gather light and then break it into spectra. To do this, the light is directed through a small entrance slit where it then goes towards a concave mirror. From the mirror, it goes on to a grating, which separates the light into its different spectral components. Finally, the light is focused by another concave mirror onto a detector, which records the signal as a wavelength. The detector records the signal by converting the photos to electrons that are digitized and sent to a computer. The software analyses the linear dispersion and number of pixels (electrons) to find the wavelengths in the spectra.10
A kind of spectrometer used for space exploration is a mass spectrometer. These work to detect and quantify the types of ions present by accelerating them through a magnetic field and/or electric field and allowing them to hit detectors. Using computers, the information can be graphed to figure out the abundance, mass, and charge of the ions.10

Example image of a data cube with the photo taken (being in this case of a coastline) overlain. The missing cross sections are the absorption lines of water.19
Courtesy of NASA/JPL-Caltech
Imaging spectrometers take photos like a camera but the pixels have multiple intensities and wavelengths. This forms 3D graphs of the wavelength related to the distance called a data cube. Each square layer of the cube represents a wavelength, with each square being a photo of the scene. Missing wavelengths are represented as missing cross sections in the cube represent absorption lines, or wavelengths of light absorbed by the gases that the light has passed through, and certain things it has reflected off of. Traditionally, spectrometers formed spectra from point sources of light by spreading out their rainbow of colours with a prism; however, imaging spectrometers accomplish a more complex version of the same task using digital methods.10
Spectrometers have been used in space missions in the past. Viking 1 and 2 in 1976 used mass spectrometers to analyze the composition of Mars’ atmosphere. Also, the Phoenix spacecraft used mass spectrometers to study the history of water on Mars. The CRISM, which is on the MRO, is an imaging spectrometer that can detect wavelengths from 362 to 3920 nanometers. The iron-rich soil of Mars makes it even easier for the CRISM to identify the absorption spectra of various minerals on Mars’ surface.9
The Evidence
So how exactly was the MRO able to detect liquid water? Scans done by the MRO and past evidence collected by other Mars orbiters have gathered evidence that hydrated salts exist on Mars’ surface. The dark streaks we have been studying on Martian soil for years have been identified as water.
The MRO began in 2006 to use its imaging spectrometer to find hydrated salts (perchlorates) responding to temperature changes. Recurring slope lineae (RSL) were found, possibly made by water flow. These streaks “flow” at temperatures above -23 degrees Celsius. The hydrated salts reduce the freezing point of a liquid brine. This is similar to the process of salting roads on Earth, as this causes ice and snow to melt more rapidly.7 Scientists combined the MRO images and spectrometer findings to find that salt was present only when the streaks were wider. The salts are probably magnesium perchlorate, magnesium chlorate, and sodium perchlorate.11 When Mars gets warm enough, these dark streaks appear on slopes on its surface. These have long been theorized to be brine flows but no evidence prior to this mission has ever been found that they contain water or hydrated salts.7
The RSL are “finger-like features” 0.5-5 m wide on 25-40 degree slopes of rock. They appear during spring and summer from 48 degrees S to 32 degrees S latitudes, favoring equator-facing slopes—times and places with peak surface temperatures from ~250-300 K”.13 They then fade in winter before returning the next spring. Some slopes are 4000 feet long. Scientists are guessing that when the temperature warms, the water flows down the slopes, and the mineral content keeps the water from freezing.12
Using the CRISM on the MRO, researchers detected spectral evidence at four different locations where the dark streaks were present. This indicates that these are brine flows and that liquid water exists on Mars.7 The reason this evidence has never presented itself before is that previous attempts had focused on taking the average spectra of larger areas, while this team focused only on areas high in RSL and in seasons when moisture in the atmosphere wouldn’t interfere or even dehydrate the salts.7
These dark streaks occur because the salts lower the melting point of water enough to create temporary downhill flows of liquid water.7 The narrow nature of some RSLs indicated that many of them can’t be liquid water directly but rather hydrated salts that result from its presence.7
The CRISM, while capable of detecting the absorption lines in the Infrared spectrum that would indicate light reflecting off of hydrated salts, had a hard time detecting it, as from its view the RSL often did not fill up a single pixel. However, by carefully extracting single pixels which contained mostly RSL and reducing uncertainties, the team obtained spectra that indicated hydration far above interference ranges.7
While we know they are water, the origin of RSL is still a mystery. Ice on Mars near the equator seems unlikely, and its forming from water vapor also seems unlikely.7
Another recent discovery indicating the presence of water has to do with clays. Hydrated minerals formed when rocks are altered by the presence of water have been found.14 These minerals, found in locations not created by impacts, are supposed to be more recently formed than previously thought, as they figure that clay in impact craters was brought up by the impact and so is older. 265 of 633 crater peaks studied seemed to contain hydrated salts.14
Minerals like phyllosilicates, sulfates, hydrated silica, carbonates, and zeolites were all found in the clays. Researchers hypothesize that there was water here, because that is how the clays would have been formed from dust. This discovery may indicate habitable conditions in the past, meaning there may have been life, and could potentially give scientists clues in the future as to how that life ended. “Authigenic” clays means they were formed where they are found, not brought up, so they are more recently formed than previously assumed.15
Implications
The presence of water helps us determine the viability of settling on Mars, the possible presence or past presence of microscopic lifeforms, and whether the fate of Mars could happen to Earth. “Determining whether liquid water exists on the Martian surface is central to understanding the hydrologic cycle and potential for extant life on Mars.”7
Scientists have to be careful when examining for life, and can’t go directly to the source, because microorganisms can travel on spacecraft and not die. If a rover goes right to the streaks and examines them, it might bring those organisms and earth life would be detected rather than life on Mars. The Outer Space Treaty of 1967 governs how careful people have to be when examining things in space.16
The European Space Agency has created the ExoMars program to explore Mars and the possibility of life. Two missions are planned, one in 2016 and the other in 2018. Both are to demonstrate new technologies, such as the movement of a rover. These missions will also involve learning more about the environment of Mars, including its atmosphere and the variation of water. Finally, the ultimate goal of discovering signs of life will not be abandoned.
References
1N. Taylor Redd, Space.Com (2015).
2Science.Nasa.Gov (2016).
3P. Yam, Scientific American (2001).
4M. Carr and J. Garvin, Nature International Weekly Journal Of Science 412, 250-253 (2016).
5Mars.Nasa.Gov (2016).
6F. Chou, NASA/JPL (2016).
7L. Ojha, M.B. Wilhelm, S.L. Murchie, A.S. Mcewen, J.J. Wray, J. Hanley, M. Massé, and M. Chojnacki, Nature Geoscience Nature Geosci, 8, 829–832 (2015).
8Nasa.Gov (2016)
9D. Turney. Crism.Jhuapl.Edu (2016).
10University Of Arizona College Of Optics Archive Site (2016).
11G. Webster, Mars.Nasa.Gov (2015).
12G. Webster, jpl.Nasa.Gov (2013).
13A. McEwen, L. Ohja and C. Dundas, Uahirise.Org (2016).
14Stacy, News.Brown.Edu (2016).
15V. Sun and R. Milliken, J. Geophys. Res. Planets 120 (2015).
16K. Chang, NY Times (2015).
17Wikimedia Commons (2006)
18Nasa/JPL.Youtube.(2016)
19AIRVIS.Nasa/JPL.Gov (2010)