By Connor Berglof, Anuj Brahmania, Quinn Herman, Andrew Phillips and Julianna Pickering
Introduction

Fig. 1. Plot of exoplanet discoveries according to year of discovery, colour-coded by method.1
Credit: NASA/IPAC
Until relatively recently in human history, our observational capacity from Earth has been rather limited. Presently technological advancements and innovations have arisen allowing us to uncover and understand more of the mysteries and intricacies of the universe. Recent observational research initiatives have led to the discovery of planets outside our solar system, known as exoplanets. These discoveries have raised many questions including whether or not any planets would be capable of supporting life, which has fueled motivation for additional research. This is a great example of the natural progression of scientific discoveries leading to additional inquiries and further research as demonstrated through the scientific method.
In order to fully comprehend how it is we are able to know that there are planets outside of our own solar system, we must consider how scientists discover them. Several techniques have been developed to detect exoplanets, including the radial velocity method, transit photometry, direct imaging, and gravitational lensing. To better understand the observational techniques as well as their advantages and disadvantages, these methods are discussed in the order of their development and successful implementation. Figure 1 gives a sense of the accomplishments of the methods used so far.
The first confirmed discovery of a planet orbiting a regular star occurred in October 1995 using the radial velocity method.2 In more recent years the extremely successful NASA Kepler Space Telescope mission, which uses the transit method, has been able to continuously monitor over 150,000 stars beyond our solar system and to date has provided scientists with over 1,000 verified planets with even more candidates to consider for further study.3
Radial Velocity (Doppler Spectroscopy)
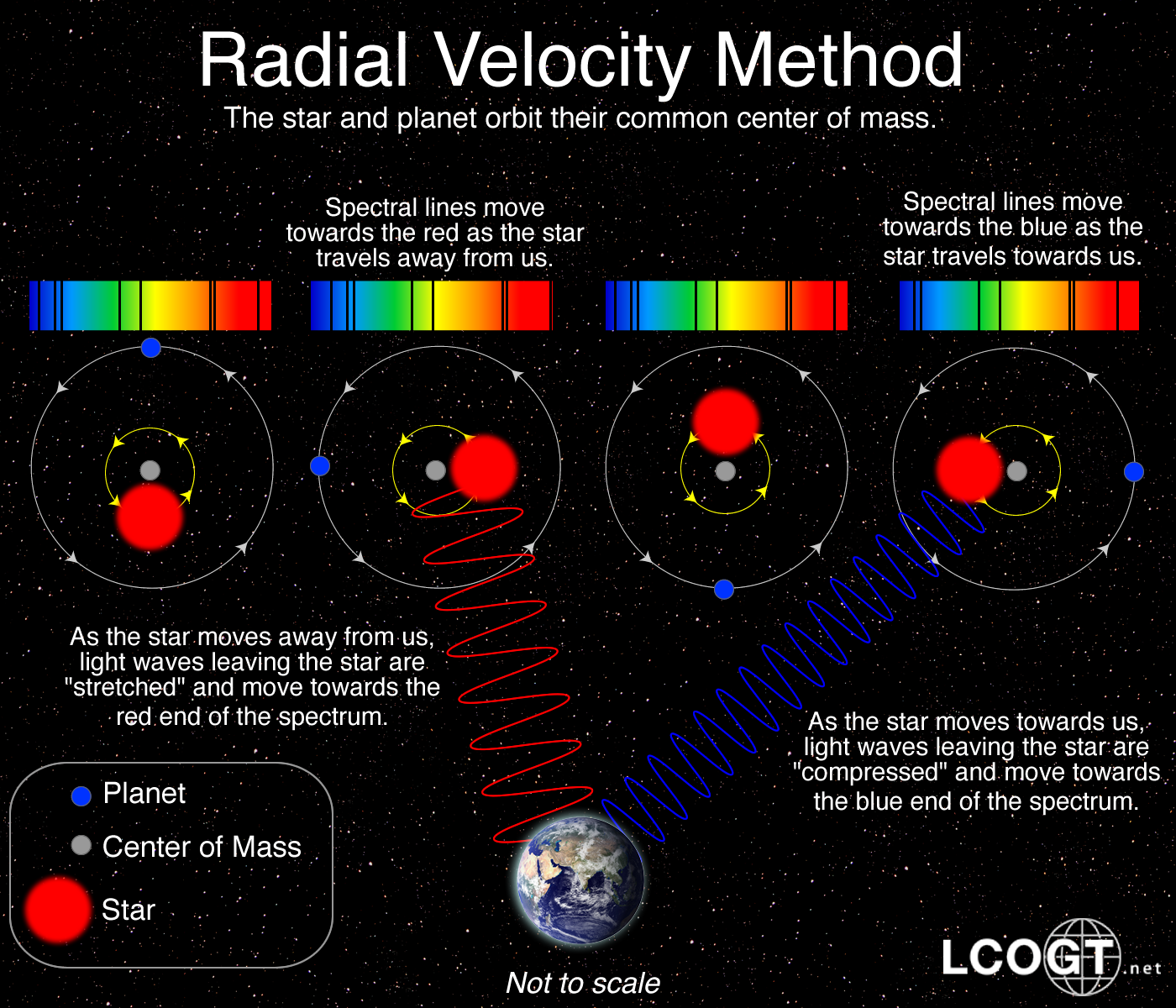
Fig 2. Doppler shifts observed using the radial velocity method of detecting exoplanets.6
Credit: Las Cumbres Observatory Global Telescope Network.
As Figure 1 shows, until recently the radial velocity method was the dominant technique used to detect exoplanets and was in fact the original method of locating exoplanets.4 The radial velocity method is based on the observation that any star orbited by a planet is subject to the gravitational pull of the orbiting planet, which in effect causes the star to move. The movement is very slight and follows a small circle or ellipse pattern causing the star to wobble forwards and backwards periodically. The small movements are observed as shifts in the star’s light spectrum or colour signature and are monitored by spectrographs on Earth or via space telescopes. These small shifts in the spectrum are actually the apparent change in the wavelength of the light due to a well-known phenomenon called the Doppler Effect.5
As you can see from Figure 2, when the star moves away from us on earth, the light waves emitted by the star are stretched and therefore move toward the red end of the spectrum, which is referred to as red shift. Conversly, when the star moves towards us on earth, the light waves emitted by the star are compressed and therefore more towards the blue end of the spectrum, which is called blue shift. The spectrographs used to detect these changes are extremely sensitive with some instruments able to detect velocities as low as 1 meter/second.7 If the star exhibits periodic red and blue shifts over a period of time, astronomers infer that the star is moving due to the gravitational force from another object or objects.
The radial velocity method has been one of the most successful detection methods historically. It has been responsible for the discovery of hundreds of exoplanets.8 The degree of movement detected using this method can also reflect the mass of the planet orbiting the observed star. Scientists are sometimes able to determine the orbiting planet’s density and composition when the planet’s radius can also be inferred through the transit method.7
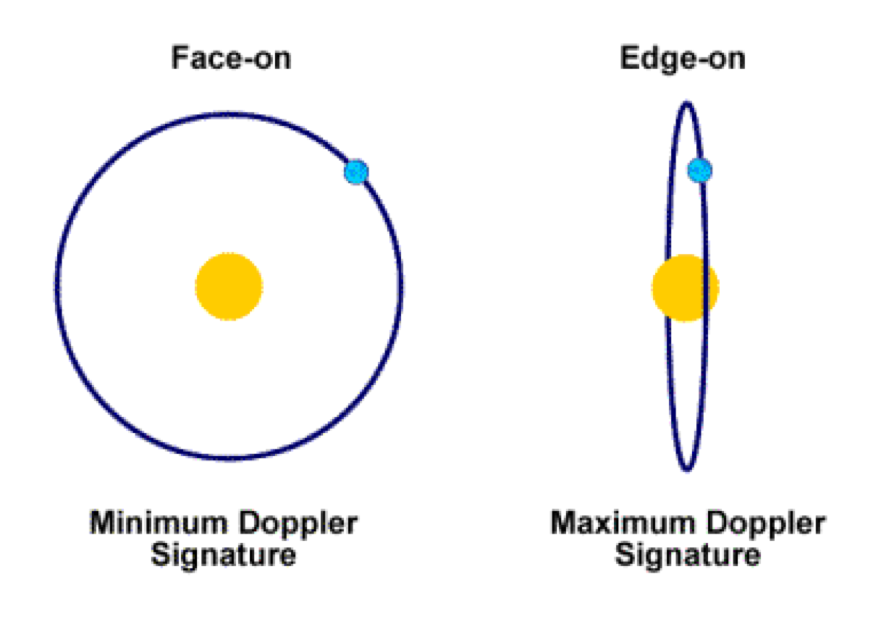
Fig 3. Sensitivity of Doppler shift determined by orientation of orbiting planet
Credit: Hugh Osborne.
Although it is a very effective tool and was the first method to successfully detect exoplanets, the radial velocity method also has limitations. For instance, the movement of the star being observed has to be parallel to earth since only the portion of movement directly toward or away from Earth can be detected. If the exoplanet orbits the star in a face-on orientation, no signature will be received at all.9
The radial velocity method can also only detect planets about 100 light years away from earth since the observed light, broken down into it’s spectrum, spreads out and makes it harder to detect. This is less of a problem for large telescopes, but such instruments can be very expensive and hard to come by as there are only a few and they are sought after by astronomers for many other applications.
Direct Imaging
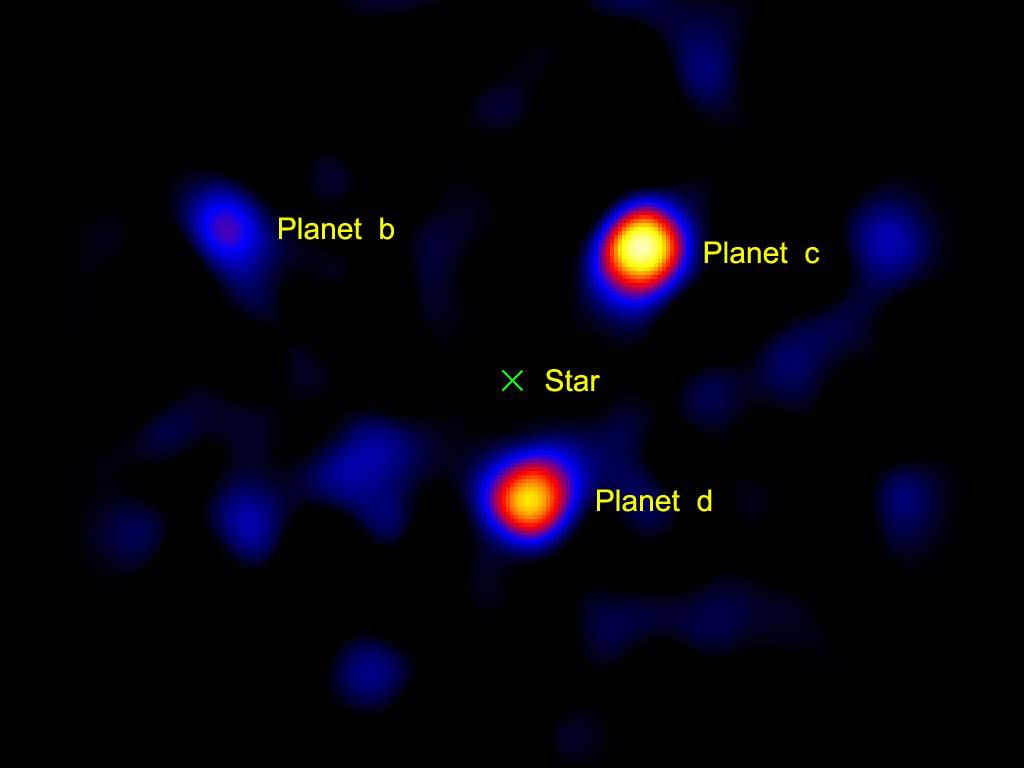
Three planets, likely gas giants like Jupiter, 120 light-years away directly imaged orbiting the star HR8799 (the light of which is being blocked by a coronagraph).10
Credit: NASA/JPL-Caltech/Palomar Observatory
Direct imaging is very simply put, observing exoplanets with an exceptionally high-tech telescope. Normally due to the faintness of the planets themselves, they are very difficult to observe optically. Some exoplanets however can be imaged in the infrared range of the spectrum as they are still retaining heat left from their formation. The heat is emitted as thermal radiation, which registers in the infrared range.
For most exoplanets however, this is not possible. Due to the limitations of this method of detection, this technique primarily produces discoveries of young planets with large masses.8 In most cases, the small and dim planets are lost in the overpowering glare of the stars they orbit.11 One way of overcoming the excess light is by using a coronagraph, which uses an object called an occulting spot, or focal plane mask, placed between the subject and the observer, to absorb most of the light from the centre of the field of view so that the light from the central source is concentrated around the edge of the telescope pupil. The next phase of the coronagraph, the Lyott stop, then blocks the remaining rings of light while allowing most of the light from the surrounding sources to pass through to the final image.12
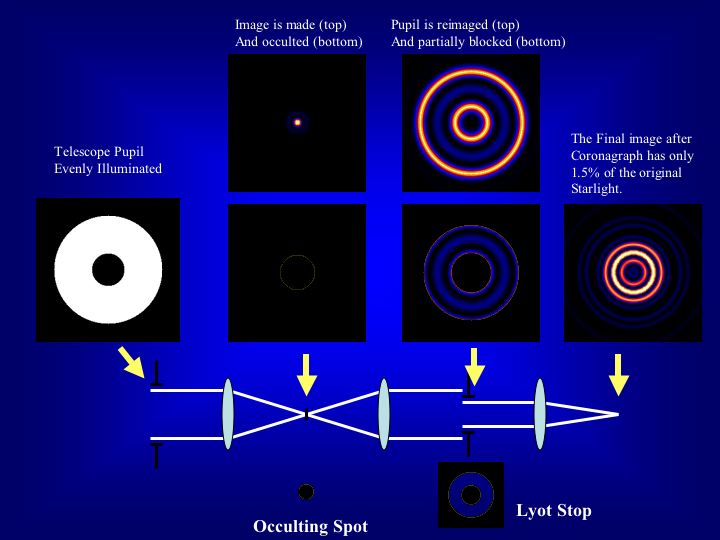
Fig 5. How a Lyott coronagraph works.
Credit: Ben R. Oppenheimer.
This technique was originally designed to allow view of the heat rings around the sun. For the purpose of detecting exoplanets, this technique may render previously washed out exoplanets visible allowing the direct imaging method to be more productive in detecting exoplanets.
Direct imaging is not be the most effective way of observing exoplanets. However, being able to see the planets rather than merely detecting their presence has a certain novelty all its own. Furthermore on the few occasions when direct imaging works, it can provide scientists with information that would be impossible to get any other way. For example, Fomalhaut b, which when observed is very dim on the infrared spectrum but remarkably bright optically, led scientists to theorize that due to the planet’s extreme orbit it is actually surrounded by a ring of dust which is actually reflecting starlight.13

Fig 6. Image taken with the Hubble Space Telescope shows the orbital motion of the planet Fomalhaut b. Astronomers have calculated that the planet is in a 2,000-year-long, highly elliptical orbit. The black circle at the center of the image blocks out the light from the bright star, allowing reflected light from the belt and planet to be photographed.13
Credit: NASA/ESA
In addition to its at times cumbersome detection efficacy, direct imaging often produces distorted images. The distortion can be caused by temperature differences that cannot be alleviated even with the use of a coronagraph or disruption from atmospheric turbulence. More recently, advancements in adaptive optics have been developed to mitigate such interferences12 and functionality of the method can improve when used via the Hubble Space telescope, but this inevitably makes it more expensive. Without the aid of a coronagraph, direct imaging is not a good candidate for large-scale surveys searching for new exoplanets.
Transit Photometry
Transit photometry detects distant planets by measuring the slight dimming of a star as an orbiting planet passes between it and the Earth. If this dimming is detected consistently—at regular intervals and last a fixed period of time—astronomers can claim to detect an exoplanet. This method can also be used to determine other information about the planet, such as size and even the chemical composition of that planet’s atmosphere.14 The degree of the dimming can be used to determine the size of the planet based on the area of starlight that the planet blocks. The atmospheric composition is determined by analyzing which wavelengths of light are absorbed by the exoplanet’s atmosphere.14
While transit photometry can be used to determine a considerable amount of information—and is in fact the most sensitive method for detecting exoplanets14—there are a few substantial disadvantages to the process. Primarily, it relies upon a transit. These are incredibly rare and the majority of planets will never actually cross between their star and Earth. This is due to the orbit of the exoplanet around its star. Only a specific type of orbit—called edge-on—can be detected. An edge-on orbit is where the planet crosses between the viewer (from earth) and its star. It has been estimated that merely 1-2% of all exoplanets have an edge-on orbit.15
In order to gather any information, any transit (which are often short) must also be witnessed—which requires constantly monitoring the sky waiting for a transit. It is also impossible to draw any meaningful conclusions from a transit—at least three must be observed to conclude that the object in question is actually a planet and not some other astronomical phenomenon. Many phenomena observed by transit photometry are eventually determined to not be exoplanets.
Gravitational Lensing

Fig. 8 Diagram which shows light originating from a source star and being bent by a lens star as the light travels to Earth.
Credit: Grant Christie.
Gravitational lensing is based on Einstein’s general theory of relativity. One of the consequences of the theory is that as light travels past a celestial body, that body’s gravity will bend the light ever so slightly. Gravitational lensing applies this to detect an increase in the brightness of a distant star incredibly far away from Earth that a planet happens to pass in front of. The process involves two stars—one far away (the source star) and one closer to Earth (the lens star)—and a planet which orbits the lens star. If these two stars line up from the perspective of Earth, the light emitted by the source star will be bent by the lens star.
As the two stars form a perfect line, the brightness that we observe through a telescope is amplified. Telescopes allow us to see clearer images because they a higher resolving power than the human eye. A high resolving power allows an observer to see finer detail because more information (light) is packed into a smaller area. This condensing of light does not, however, allow an observer to differentiate between two different sources of light. As a result, the only image we can see is that of amplified brightness. In some cases, the light ‘from’ the lensing star is amplified by up to 1000x. This lasts until the lensing star and the source star lose alignment, which happens naturally as galaxies both move and rotate in the universe. The lensing which can take anywhere from a few weeks to a few months.15
During the alignment of the lens and source stars, light bends around the lensing star two distinct streams of light reach Earth—the light originating from the source star and from the lens star. But as light also travels past the planet, another stream of light appears. Keep in mind that light is a type of energy, we cannot physically ‘see’ the light that arrives at Earth, but we can detect when these streams of light are combined because their brightness appears amplified. What is important is that we can detect when the light is being bent by the lens star and when it is being bent by the exoplanet. The light bent by the planet appears to us as a temporary spike in brightness. It may last for a couple of hours or multiple days. The duration and intensity of the planet’s bent light can be used to be used to determine a planet’s mass, orbit, and period with a high accuracy and probability.16
Like all methods of discovering exoplanets, gravitational lensing has inherent advantages and disadvantages. It can be used to discover planets incredibly far away from Earth—sometime thousands of light years away.16 Since gravitational lensing boils down to setting up a large telescope and watching for changes in flux measured, it is possible to examine massive search areas. We are essentially targeting tens of thousands of stars at the same time. However, the nature of the method has specific draw backs, particularly concerning repeatability and the accuracy of measurements. Due to the particular conditions that need to be met (that a lens star, with a planet, and source star line up from our perspective on Earth) to detect an exoplanet through gravitational lensing, it is unlikely to repeat these observations after stars fall out of alignment. If scientists’ measurements are even slightly inaccurate any error is greatly amplified and the results will not correspond to reality in any meaningful capacity.
Conclusion
Gravitational lensing, radial velocity, transit photometry and direct imaging are the most common methods used to detect exoplanets in the twenty-fitst century. These four processes all have major strengths and weakness and can sometimes be used to complement each other in the search for exoplanets. For example, radial velocity gives mass, transit gives size, so combining we get density, from which we can infer physical properties such as the chemical composition. In the quest for discovering more about the universe, each method gives us a piece of the greater image. Currently, we are finding small pieces of a remarkably vast universe and will no doubt bear witness to even more extraordinary discoveries in years to come.
References
1NASA Exoplanet Science Institute, NASA Exoplanet Archives, WWW document, (http://exoplanetarchive.ipac.caltech.edu/exoplanetplots/).
2D.E. Gary, Search for Extrasolar Plaents, WWW document, (https://web.njit.edu/~gary/320/Lecture10.html).
3F. Chou & M. Johnson, NASA’s Kepler Marks 1,000th Exoplanet Discovery, Uncovers More Small Worlds in Habitable Zones, WWW document, (http://www.nasa.gov/press/2015/january/nasa-s-kepler-marks-1000th-exoplanet-discovery-uncovers-more-small-worlds-in).
4The Planetary Society. Radial Velocity, WWW document, (http://www.planetary.org/explore/space-topics/exoplanets/radial-velocity.html).
5B. Mattson, Doppler Shift, WWW document, (http://imagine.gsfc.nasa.gov/features/yba/M31-velocity/Doppler-shift-2.html).
6Las Cumbres Observatory Global Telescope Network, Radial Velocity Method, WWW document, (https://lcogt.net/spacebook/radial-velocity-method/).
7J. Stromberg, How do Astronomers Actually Find Exoplanets?, WWW document, (http://www.smithsonianmag.com/science-nature/how-do-astronomers-actually-find-exoplanets-180950105/?no-ist).
8B. Beky, Ph.D. dissertation, Harvard University, 2014, Retrieved from http://arxiv.org/pdf/1408.0401.
9H. Osborn, Kepler’s Last Stand: Verification by Multiplicity, WWW document, (http://www.hughosborn.co.uk/author/hposborn/).
10R. Whatmore, Portrait of Distant Planets, WWW document, (http://www.nasa.gov/topics/universe/features/exoplanet20100414-a.html).
11The Planetary Society. Direct Imaging, WWW document, (http://www.planetary.org/explore/space-topics/exoplanets/direct-imaging.html).
12B. R. Oppeenheimer, The Lyot Project. Coronagraphy, WWW document, (http://lyot.org/background/coronagraphy.html)
13J.D. Harrington, NASA’s Hubble Reveals Rogue Planetary Orbit For Fomalhaut B, WWW document, (http://www.nasa.gov/mission_pages/hubble/science/rogue-fomalhaut.html).
14Planetary, Transit Photometry, WWW Document, (http://www.planetary.org/explore/space-topics/exoplanets/transit-photometry.html).
15I. Waldmann, L11: Finding Exoplanets: The Transit Technique PDF document, (http://zuserver2.star.ucl.ac.uk/~ingo/Lecture_Notes_files/lect11.pdf).
16Planetary, Microlensing, WWW Document, (http://www.planetary.org/explore/space-topics/exoplanets/microlensing.html).