The Search for Exoplanets: A Habitable Suitor
By: Mason Bleakley, Aaron Gordon, Christine Koroluk, Raymond Morstad, Adam Nenson, Anthony Piska, and Cordell VanGenderen
Since humanity first turned its eyes towards the night sky and gazed in awe at the infinite wonder of the universe, we have been captivated by space. Exploration and discovery is as much a part of our human nature, as our desire to observe the depths of our origins, and understand the unknown. The search for exoplanets is one of the foremost, growing fields in the exploration of space. Exoplanets are planets located in distant extrasolar systems, orbiting stars other than our own, and vary in size from larger than Jupiter, to smaller than Earth.¹⁶ In light of the challenges of overpopulation, extreme seasonality and climate change, as well as the depletion of the world’s natural resources, something must be done. There is a new impetus to find and potentially colonize a habitable planet similar to our own. It may not be possible today, but theoretically, in the near future this may become reality. The first step is to look and learn. In this paper, we will explore some of the methods of how we have come to learn what we know today about exoplanets. Several criteria and conditions are necessary for a planet to be considered habitable. Habitable planets have a very specific range of conditions that can support life, and when searching for exoplanets, we look for similar conditions that we find on Earth. Evolution is theoretically capable of producing endless variations of life, which is another interesting aspect related to the study of exoplanets. Very few planets discovered to date have the appropriate conditions to support life, as we know it. A further consideration of the methods of discovery, and conditions of habitable features that pertain to extrasolar planets is necessary. We aim to look at which exoplanets may have the potential to support and sustain Earth-like, carbon based lifeforms. We need to be able to detect Earth size planets, orbiting Sun like stars, at distances that would support liquid water. How do we find such Exoplanets, that have the environmental conditions to support life?
Methods for Detecting Exoplanets

Figure 1: This is a figure that gives a brief history of the Kepler Space Telescope, as well as its function. Source: http://www.space.com/32850-nasa-kepler-telescope-finds-1284-alien-planets.html
Astronomers have used many different methods to discover planets beyond the solar system. It is important to note that the majority of exoplanets are of too great a distance to be viewed using traditional imaging methods from observatories. Many of the earliest exoplanet discoveries were Jupiter-sized, or larger gas giants, that were orbiting close to their parent stars. That’s because astronomers had to rely on the radial velocity technique, which measures how much the star wobbles when a planet orbits it. These large planets, being relatively close, produce a correspondingly significant effect on their parent star, causing a wobble that is comparatively easy to detect. Certain techniques of detection have recently reached their technical limit, restricting the amount of data that can be collected. The knowledge learned from the Kepler missions, combined with the application of next generation space telescopes, will allow for more detailed studies of other solar systems and the planets orbiting their host stars. Future missions will emphasize these efforts to observe more specific characteristics within individual solar systems, unveiling ringed worlds similar to Saturn, planetary moons, as well as large collections of asteroids. This would be complementary to the pre-existing models that have been created based on data (phase curves) from multiple previous missions. Observing stars, and their orbital transits for longer periods of time, is one such current objective among the astrophysics community. This will aid in improving the models based off the data from planets and stars which have already been discovered.¹
In order to find and observe these planets, scientists use various methods of observation, as exoplanets are incredibly difficult to see directly from Earth. Directly observing exoplanets, especially distant ones is extremely difficult even with advanced technology. It is therefore necessary to indirectly determine their presence. This is performed not by measuring the planets themselves, but their affect on objects nearby. The development of indirectly detecting exoplanets has vastly increased our capabilities for finding alien worlds.¹
Using data from multiple space telescopes that are fixated on certain exoplanets could tell us more about the particular features of the planet, such as atmosphere, elemental composition, and geography. As space telescopes observe stars and planets, they stream this information to Earth where computers run a series of algorithms on the data, and astronomers can then analyze the results. The culmination of multiple indirect methods being used, while detecting and observing exoplanets of interest around stars, may give greater insight into which could be a possible suitor and potentially habitable. A summary of the methods used by astronomers to observe exoplanets and stars is necessary in understanding how we know, and what we know about them today.
Transit Photometry
When it comes to finding extrasolar planets outside our own solar system, NASA’s space mission Kepler has found more than 1000 confirmed exoplanets in the last 5 years. One technique is called the Time Series Transit Photometry, which is most effective for detecting large planets in close range orbits relative to a star. The Transit Method works by detecting a routine drop in the apparent brightness of a star caused by a planet passing between the star and the Earth. This data is collected over extended periods, collecting information on all planets orbiting the star. This data is examined, and drops with routine intervals are indicative of planets. The length and intensity of the intervals can be used to determine multiple characteristics of the planet including size and distance from the star.¹ One of the main challenges with this method is that the exoplanet and the host star have to align, in a line of sight sequence when being viewed from the focal point of Earth. A majority of planets that orbit other stars will not pass in front of their star in this manner, and therefore cannot be detected using this method when relatively viewed from Earth. Another difficulty when taking into account the limits of this planetary detection method is the star’s total luminosity. During a transit, the signature ‘drop’ in apparent brightness detected is less than 0.01%, and is a result of the planets being smaller than their host stars. Considering these difficulties and technical constraints, this primarily remains one of the best methods for detecting and further studying exoplanets.This method is even capable of detecting exoplanets that are orbiting at an Earth like distance from its star but it is very difficult to detect such planets because these planets must be orbiting in a detectable plane which has a small chance of occurring, specifically, the chance is 0.43%.⁴ This observational method of detection may also be able to observe ring structures, such as the ones around Saturn or Jupiter’s moons, by measuring and using an understanding of orbital mechanics. The Kepler space telescope is one that finds exoplanets by carefully watching the star light exhibited by a solar system’s host star. Future missions such as NASA’s TESS and PLATO will also use this method.⁴
Radial Velocity
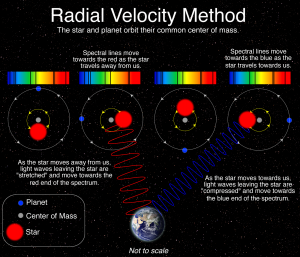
Figure 2: This figure explains clearly how the radial velocity method works. It also gives a visual representation of how this method of observation works. Source: http://lcogt.net/spacebook/radial-velocity-method
Another primary method that scientists use to detect extrasolar planets is the Radial Velocity method. Both the Transit Method and the Radial Velocity Method are the two big indirect imaging methods, which means that scientists are not directly taking pictures or images of objects that orbit other stars. With the Radial Velocity Method, we can see that as a planet orbits its host star, it initially appears that there is only a gravitational pull being exerted on the orbiting planet, while the star remains stationary. However, considering what we have come to learn about Newtonian physics and orbital mechanics, we know that the planet also produces a significant gravitational field that acts as a force pulling on the star as well, essentially causing the star to wobble slightly as the planet complete an orbit because it changes the center of mass away from the center of the star. This small yet significant wobble can be detected by telescopes and observatories on Earth as the star’s spectrum of colour shifts colour and is a result of the Doppler Effect. The Doppler effect is what causes light waves to stretch and contract as the light source moves towards or away from the observer. This change in wavelength causes the colour of stars to shift red when they are moving away from us, and shift blue when moving closer to us, as displayed in figure 2. Due to the wobbling of the star caused by the orbiting planet, the star can be pulled towards or away from Earth. When the star wobbles towards Earth, the visible light waves that it emits get stacked closer together causing the light to appear blue. As it wobbles away from Earth the light waves become more spread apart, making the visible light look red. Astronomers are able to detect exoplanets with this method by measuring the spectral fluctuations of stars. This method is currently the best for detecting big planets that closely orbit their stars.¹
Direct imaging

Figure 3: This is an animation that shows the first extrasolar planets that were discovered using direct imaging. We can see the extrasolar planets orbiting their sun in this animation. Source: https://commons.wikimedia.org/w/index.php?curid=55463078
With the right conditions, an exoplanet can be directly imaged around the star it orbits. The stars visible light will be millions of times brighter than the planets that orbit it; but when looking at the infrared light of a star system, a star is only thousands of times brighter than its surrounding planets. These differences are greater in younger star systems because these systems are still cooling which emits more infrared light than that of older star systems. When imaging a planet directly, the planet must be must at a considerable distance from its parent star so that it can be defined in the the glare of of the light from its parent star.¹
Timing Method
There are many other methods of detecting exoplanets, but they are used less frequently because they are much harder to detect exoplanets with. However, we will go through a couple of the less common methods of detection. One very successful way to detect exoplanets is through the timing method. This method, most of the time, requires the exoplanet to be orbiting around a pulsar in order for detection and therefore cannot be used regularly.¹ A pulsar is a star that emits a highly magnetic field and has an axial rotation that makes it appear like it is pulsing, hence pulsar. These pulses are highly regular and therefore can be timed very precisely. When an exoplanet is orbiting a pulsar, the timing of these pulses is altered because the gravity of the exoplanet causes the pulsar to wobble. Even though this wobble is very small, it has an effect on the timing and pulses emitted by the pulsar and the presence of an exoplanet can be inferred because of this change.¹ This method can also be used with regular stars, but it also also much less accurate. With regular stars, their luminosity fluctuates and these fluctuations in luminosity are also predictable. When the predictability pattern of these stars is altered, an exoplanet is inferred to be orbiting the star.¹
Astrometry Method

Figure 4: This figure shows how a star an wobble because of a change in center of mass with the presence of an orbiting planet. This shift causes both the planet and the star to orbit the center of mass. The large white circle represents the star, while the small circle represents the exoplanet. Source: http://www.skymarvels.com/infopages/exoplanets.htm
The astrometry method involves looking at the wobble of a star which is caused by the gravity of an orbiting exoplanet. This involves tracking the changing position of a star over time and imaging the star directly which is much more difficult than using the Doppler shift, as explained earlier in the radial velocity section, effect from the radial velocity method because it requires very precise measurement of the very slight wobble of the star, in contrast to the radial velocity method where you only have to measure a change in colour of the star. There are also many difficulties in using this method with ground based telescopes because atmospheric changes can make observing the changing position of the stars very difficult.¹ In 2013, the European Space Agency (ESA) launched a spacecraft called GAIA that is currently searching for exoplanets using this method because it avoids the atmospheric disturbances that we would observe in ground based telescopes.¹ GAIA began its work in July 2014 and has since cataloged the position and brightness of over a billion stars which gives us a tremendous amount of data to search for exoplanets.¹⁵
Gravitational Microlensing
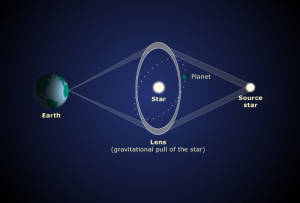
Figure 5: This figure shows how light is bent around a lens star to produce the lensing effect. Source: http://www.teara.govt.nz/en/diagram/8008/gravitational-microlensing
Gravity is something that we have seen to affect everything in our universe, this includes light. Most planets in our galaxy are too small to significantly influence light, but stars are much more massive and can have massive effects on light. We use this in order to observe gravitational microlensing. This method of detecting exoplanets consists of observing gravity bending light from behind a massive object, such as another star, and measuring this bent light.¹ When Albert Einstein published his theory of general relativity, one of the consequences of this was the prediction that the path of light could be deflected by gravity. This means that light passing a massive body, such as a star, can be bent.This method works because this bending of light is similar as to what happens with light when it passes through a magnifying glass and scientists can predict, thanks to Einstein’s theory of general relativity, how this light will bend around the star. When there is a disruption in the predicted bend of light, then scientists can infer an exoplanet is orbiting the star the lens star because the gravity of this orbiting planet contributes to the lensing effect of the lens star (figure 5). This method only works when the lens star and the observed star are perfectly lined up and these cases of microlensing are relatively rare and difficult to predict, and for this reason, this method is not used nearly as much as other methods.¹
Conditions to Support and Sustain Life (As Prescribed by Earth)
One might see the conditions to support life as being fairly straightforward. We would obviously need to be looking for a planet with a suitable atmosphere similar to Earth’s and liquid water, but in reality there are numerous other considerations that need to go into finding a planet that has all the conditions to support life. Astronomers like to refer to these ideal conditions as the ‘Goldilocks principle’, after the classic fairytale of ‘Goldilocks and the Three Bears’. The first thing we should look for when looking for an exoplanet with the ideal conditions for life, is a planet that contains the right elements and compounds that life would need to survive. These include water, oxygen, nitrogen, carbon, and hydrogen just to name a few.5 These are ingredients that all life, as we know, needs to survive.
The next thing we would look for is a planet with the right crust. The Earth has a solid crust and a molten center that creates a magnetic field that protects it from much of the sun’s harmful radiation.5 This means that we cannot live on world that is molten because the crust would be too hot, nor could we live on a gas giant, such as Jupiter, because there is no stable crust to live on.
Another very important condition we would need on a habitable planet is the right temperature. This is because, as mentioned earlier, one of the conditions for life is liquid water and if the temperature is too hot or too cold the water will either be in gaseous or solid states. Earth’s position in relation to the Sun is vital in dictating its temperature and so are the presence of proper atmospheric compounds, such as carbon dioxide, methane, and water, since they work together to create a greenhouse effect which maintains the perfect temperature where liquid water can thrive on Earth’s surface.5 Currently, Earth is situated comfortably in the Sun’s habitable zone and the atmosphere is effectively trapping the Sun’s heat, creating a situation where liquid water, and subsequently life, can exist.

Figure 6: This figure explains the Earth Similarity Index and gives a visual representation on how the planets in our solar system compare to Earth using this index. Source: http://phl.upr.edu/projects/habitable-exoplanets-catalog/methods
The presence of our Solar System’s largest planet, the gas giant Jupiter, also plays a role in maintaining habitability. Due to its massive size and gravitational pull, it has helped determine Earth’s orbital path around the Sun.5 Jupiter also acts as a shield for Earth since it absorbs many meteorite strikes. However, the massive gravity from the planet could alter the paths of other small objects, possibly sending them towards Earth.5 Overall, the effects of Jupiter have played a role in the placement of Earth in the habitable zone of our Solar System as well as the general protection of Earth from stellar bombardment, contributing to Earth’s habitability and longevity as a planet.
As we can see, there are many conditions to take into account when looking for a habitable exoplanet and it may be very rare to find one with all the right conditions. According to Earth’s standards, the habitability of an exoplanet is generally reliant on “plate tectonics, a global magnetic field, a hydrosphere, and the distance of the habitable zone from its host star”.5 Although the conditions for a perfectly, Earth-like planet are quite extensive and specific, the search for such a planet continues. And in the grand scope of the known universe, it’s only a matter of time until more Earth-like planets, and possibly other signs of life, are discovered.
Possibly Habitable Exoplanets
Using the described technology and criteria several planets have been discovered meeting the habitable criteria. The closest potentially habitable planet is Proxima Centauri B located around the star Proxima Centauri 4.2 light years away. The planet is likely to be tidally locked to the star and larger than Earth.⁷ While this does cast doubt on its habitability, it is still possible for the planet to be habitable. Since its discovery in 2014 using the Kepler Spacecraft, Kepler-186f has held the title of most likely to be habitable. Its orbit places it nicely it its habitable zone (unlike many other potentially habitable planets that are nearer to the edge). More importantly though is Kepler-186f’s mass. Weighing within 10% of Earth’s mass, it is the closest exoplanet in comparison with the Earth, excluding the most recent discoveries (that we still don’t know much about).⁸ Besides knowing the planet is rocky, the chemical composition of Kepler-186f has not yet been determined and therefore it is unknown if the planet is truly habitable. There has also been a recent discovery earlier this year regarding habitable planets. In a press conference on February 22 NASA announced the discovery of a seven-planet system dubbed TRAPPIST-1. The system, a mere 40 light years away was discovered by the Spitzer Space Telescope. Three of the planets of Earth size have been determined to be within their star’s habitable zone, and are believed to be rocky planets. Due to the recentness of the discovery not much else is known about the planets.⁹ There are multiple other exoplanets out there that have been discovered that could be considered habitable, and there are likely many more yet to be discovered.

Figure 7: This figure shows all the discovered exoplanets as of 2014. Only the the planets that are less than 10 Earth masses are labeled. The green shaded areas are the potential habitable zones and the size of the circles in the figure corresponds to the radius of the planets. i.e Larger circles have a larger radius. Source: http://phl.upr.edu/projects/habitable-exoplanets-catalog
Conclusion
The discovery of exoplanets is significant through the unveiling of the specific details that are vital in determining their habitability. The nature of these details, such as atmosphere, geographical features of the planet, and seasonality, along with other significant findings, could potentially be produced if a number of factors were to be considered and implemented. The first of these is the study and development of new observational methods and techniques. Additionally, the number of astronomers and scientists dedicated to using these techniques and analyzing the light spectrum data from these exoplanets would be critically important. Secondly, while considering the distance to other planets in other solar systems, we would need to utilize the capacity of multiple space telescopes and observatories aimed simultaneously at planets of interest. Significant resources would have to be allocated to the development of software and hardware that we would need to use to process the vast amounts of data incoming from these telescopes over prolonged periods of time. Astronomers and scientists have recently begun applying the use of artificial intelligence to help process all of the of data streaming in from space telescopes. Another factor that will greatly help the study and detection of exoplanets is the deployment of additional new-age deep space telescopes and satellites. All these tools play a major role in what we may find. Prolonged exposure by observation and the cumulative efforts of detection and techniques could potentially bare answers to some of our many questions and mysteries of the universe.
References
¹Simon Fraser University, Methods for Detecting Exoplanets,(https://www.sfu.ca/colloquium/PDC_Top/astrobiology/discovering-exoplanets/exoplanet-detection-methods.html)
²E. Howell, Exoplanets: Worlds Beyond Our Solar System, (http://www.space.com/17738-exoplanets.html)
³J. Stromberg, How Do Astronomers Actually Find Exoplanets?, (http://www.smithsonianmag.com/science-nature/how-do-astronomers-actually-find-exoplanets-180950105/)
⁴Simon Fraser University, Kepler Space Telescope, (https://www.sfu.ca/colloquium/PDC_Top/astrobiology/discovering-exoplanets/why-do-we-need-telescopes-in-space-/Kepler.html)
⁵Robert Lamb, What is it about Earth that makes it just right for life?, (http://science.howstuffworks.com/life/evolution/earth-just-right-for-life.htm, 2010)
⁶E. Howell, How Mighty Jupiter Could Have Changed Earth’s Gravity, Space Daily 20, (http://www.astrobio.net/news-exclusive/how-mighty-jupiter-could-have-changed-earths-habitability/, 2014)
⁷P. Brennan, ESO Discoveres Earth-Size Planet in Habitable Zone of Nearest Star, (https://www.nasa.gov/feature/jpl/eso-discovers-earth-size-planet-in-habitable-zone-of-nearest-star, 2016)
⁸J. Culler, Kepler-186f, the First Earth-size Planet in the Habitable Zone, (https://www.nasa.gov/ames/kepler/kepler-186f-the-first-earth-size-planet-in-the-habitable-zone, 2014)
⁹E. Landau, NASA Telescope Reveals Largest Batch of Earth-Size, Habitable-Zone Planets Around Single Star, (https://www.nasa.gov/press-release/nasa-telescope-reveals-largest-batch-of-earth-size-habitable-zone-planets-around, 2017)
¹⁰Open Exoplanet Catalogue, (http://www.openexoplanetcatalogue.com)
¹¹Nasa, 5 Ways to Find a Planet, (https://exoplanets.nasa.gov/interactable/11/)
¹²Exoplanet Detection Techniques, (http://www.mpia.de/homes/ppvi/chapter/fischer.pdf)
¹³Detection and Characterization of Exoplanets and Disks using Projections on Karhunen-Lo`eve Eigenimage, (https://arxiv.org/pdf/1207.4197.pdf)
¹⁴Iflscience, Water Found on the First Exoplanet we Discovered, (http://www.iflscience.com/space/water-found-on-one-of-the-first-exoplanets-we-ever-discovered/)
¹⁵ESA, GAIA’s Billion-Star Map Hints at Treasures to Come, (http://www.esa.int/Our_Activities/Space_Science/Gaia/Gaia_s_billion-star_map_hints_at_treasures_to_come )
¹⁶Harvard-Smithsonian, The Mearth Project, (https://www.cfa.harvard.edu/MEarth/Science.html)