Mercury
Authors: Erik Andvaag, Paige Hirschfeld, Lacey Manley, and Lara Paul
1.0 Introduction
Mercury, one of the ancient planets, has been observed since time immemorial. However, despite this legacy, and despite lying closest to the Sun, the true nature of this small, barren world remained shrouded in darkness until very recently. By examining the history of telescope observations, the Mariner 10 mission of the 1970’s, and the recent MESSENGER mission, this project will provide an overview of what has been learned about the geological makeup of Mercury in the past half century. A deep understanding of Mercury’s geology is of great importance for several reasons. Firstly, it has allowed for accurate calculations of planetary density, mass, and volume. It has also helped astronomers infer what geological processes are currently operating on its surface, and which processes may have been important in the past. In addition to providing a better picture of its own formation, a number of discoveries have also been useful for understanding the Solar System at large, and Earth’s place among the planets. By exploring Mercury, astronomers have gained greater insight into the conditions of the early inner Solar System,1 have improved their knowledge of planetary magnetic fields,2 and have expanded their search parameters for the presence of water on extraterrestrial and extrasolar planets.3 The spotlights of Mariner 10 and MESSENGER have at long last begun to illuminate Mercury’s long-held secrets.
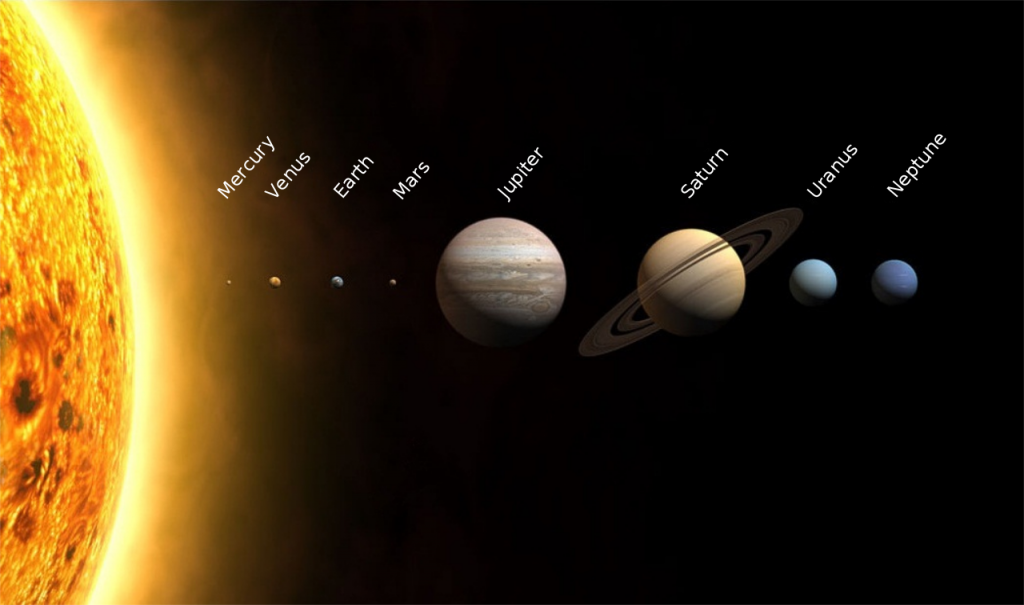
Figure 1: A representative image of the Solar System and the planets with sizes to scale, but not distances. Credit: NASA
2.0 Telescope Observations
2.1. Challenges for Earth-based Observation and Basic Physical Data
Before the recent dawn of the space age, humanity’s knowledge of Mercury was limited to what could be learned from observing the planet in the terrestrial sky. Unfortunately, Mercury presents numerous challenges for Earth-based observation which the other planets do not. Due to its proximity to the Sun, Mercury can only be seen in the sky during dawn or twilight from Earth. Most space telescopes, including the Hubble Space Telescope, cannot be aimed towards the planet at all because their sensitive equipment may be damaged from the intensity of the Sun’s light.4 Additionally, because it only appears close to the horizon, light reflected from Mercury’s surface must travel through a long air path, which minimizes the contrast of surface features and affects image quality due to atmospheric turbulence.5 A final complication is that the angular extent of Mercury’s disk appears very small from Earth, which causes poor image resolution. Despite the host of issues, astronomers were able to deduce a few of Mercury’s basic physical characteristics before the launch of any spacecraft. The two most fundamental features known to astronomers at the time were: (1) relative to the other planets, Mercury was unusually dense, and (2) its surface was likely cratered and very similar to Earth’s Moon.6 Determining Mercury’s density was fairly straightforward. First of all, the planet’s mass was learned by analyzing its gravitational effect on other objects in the inner Solar System, such as planets, asteroids, and spacecraft.5 The radius of Mercury was then determined from photometric observations during transits of the Sun, as well as radar reflection measurements.5 From these values, Mercury’s unusually high density was discovered, which suggested an internal composition of 65 to 70 percent by weight of metallic material (believed to be iron), and 30 percent by weight of silicate material.7 Determining the nature of Mercury’s surface would prove to be a more involved undertaking.
2.2. Early Attempts at Surface Mapping
Knowledge of Mercury’s topography was admittedly rudimentary prior to Mariner 10’s photographic mapping. The first attempt to map the planet’s surface was made by Johann Schröter (1745 – 1816); however, his sketches were mostly inaccurate.8 Several other mapping attempts were made in the late 19th and early 20th centuries, including efforts by Giovanni Schiaparelli (1835 – 1910), Percival Lowell (1855 – 1916) and Eugene Antoniadi (1870 – 1943) (Figure 2).9 Surface features were frequently proposed which would later prove to be illusory, such as Lowell’s description of canals similar to those which were at the time thought to exist on Mars, and Antoniadi’s supposed observation of dust clouds. Furthermore, due to the incorrect belief that Mercury’s axial rotation and revolution shared a periodicity of 88 days, many features were mapped incorrectly, working under the assumption that the same hemisphere of Mercury always faces Earth.8, 10
2.3. The Moon: An Analogue?
In the 1960s, photometry and reflected radar signals indicated that Mercury shared highly similar surface properties with the Moon.5 Because the Moon’s surface was believed to be an effective analogue, information gathered from the Apollo program concerning the lunar surface was extrapolated to Mercury, after adjusting to fit the known general composition of the planet. For example, the presence of minerals such as plagioclase, pyroxene, and
ilmenite was inferred from lunar samples, but it was also understood that heavy metals, particularly iron, would be present in higher ratios on Mercury’s surface in order to be compatible with the planet’s high
mean density.5
However, even though most astronomers believed Mercury’s surface would be similar to the Moon, it could not be definitively determined until Mariner 10 returned the first high resolution images of the surface.7 Beyond the confirmation of their general similarity, the photographs also revealed subtle differences in topography and composition between Mercury and the Moon (Figure 3). The most prominent topographic difference was Mercury’s lack of the expansive lava plains which characterize the Moon’s surface (maria).11 Closer inspection of images also showed Mercury’s surface to be marked by large curved cliffs (termed lobate scarps); these structures are not seen on the Moon, and are currently believed to have been created as Mercury cooled.12 Cooling caused the planet to shrink, which broke the crust and formed these cliffs. Finally, although Mercury and the Moon are both largely grey in appearance, images which were manipulated to exaggerate colours showed slight differences in hue, a clear indication of compositional differences between these two bodies.11 Taken all together, the many small differences in topography and composition indicated to astronomers that, despite both cooling from liquid rock, Mercury and the Moon could not have formed in precisely the same way. Only up-close photographs were able to provide astronomers with the level of detail necessary to perceiving these subtle distinctions. The images returned by Mariner 10 would forever change humanity’s perception of Mercury.
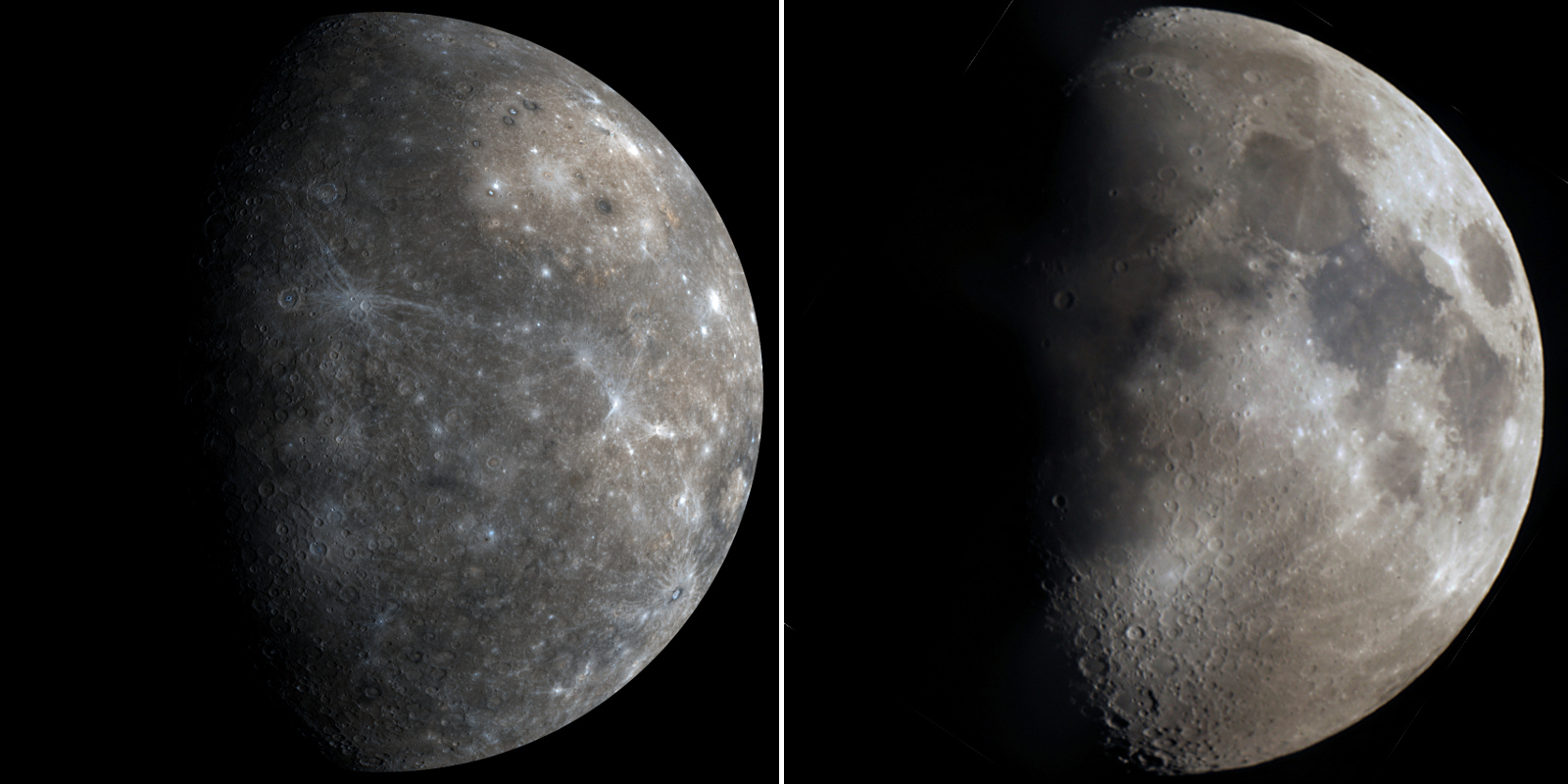
Figure 3: “Spot the Differences”: Mercury (left) and Earth’s Moon (right). Credit: NASA/Johns Hopkins University Applied Physics Laboratory/Carnegie Institution of Washington (Mercury) │ Eric Kilby (Moon) │ The Bruce Murray Space Image Library (comparison image)
3.0 Mariner 10 Mission
3.1. Mission Objective
The objective of the Mariner 10 mission was to photograph Mercury’s surface, discern if the planet had a magnetic field, and measure the characteristics of the surface and the atmosphere.13 The Mariner 10 spacecraft was part of the Mariner series that was launched by NASA in 1962. Mariner 10 was the last of the ten spacecraft that NASA launched to explore Mercury, Venus and Mars.13 This was the first spacecraft to visit Mercury and would be the first to use another planet’s gravity to change its trajectory. This technique would prove to be very important in future space missions.13 Mariner 10’s mission to the planet was to be a success, as it returned the first images of the surface, giving scientists their first close-up look of our Solar System’s innermost planet.13
3.2. Mission Details
The Mariner 10 mission type was a flyby. It did three flybys, the first on March 29, 1974 at a distance of 703 km, the second on September 21, 1974 at a distance of 48,069 km, and a third on March 16, 1975 at a distance of 327 km.13 The mission launched on November 3, 1973 from the United States.14 The mission ended on March 24, 1975, and it is estimated that Mariner 10 is still orbiting the Sun.13
Spacecraft Instruments (Figure 4):
1) imaging system – this instrument imaged the surface of Mercury, providing scientists with the first images of Mercury taken by a spacecraft.15
2) infrared radiometer – measured the surface temperature without having to touch the surface. The instrument measured the temperature changes on Mercury and the extreme changes from day to night that the planet faces. The resolution was as fine as 45 km/pixel. The temperature bands measured were used to infer the roughness of the surface, size of material on the surface, and if there were rock outcrops on the surface.16
3) ultraviolet airglow spectrometer – one of the two spectrometers on the spacecraft that was used to determine the composition of the atmosphere of Mercury. It measured the emission rates of He, H, A, Ne, O and C to determine what was present in the atmosphere and radiating from Mercury.15, 16
4) ultraviolet occultation spectrometer – the second of two spectrometers that were used to determine the makeup of Mercury’s atmosphere.15, 16
5) two magnetometers – measured the magnetic field of the planet. It determined that the planet has an active magnetic field and that therefore the core must be moving.15
6) charged-particle telescope – used to detect high-energy particles. The data collected further confirmed what the plasma analyzer and magnetometers had already measured about Mercury’s magnetic field.15
7) plasma analyzer – used to measure the positive ions and electrons that were in the atmosphere and that made up the solar wind and how that wind interacted with the planet. If scientists could find out how the solar wind behaved with Mercury they would be able to determine if the magnetic field was similar to the one that is on Earth. The data that they collected supported the data that was collected from the magnetometers.15, 16
3.3. Mission Findings
The first images (Figure 5) returned by Mariner 10 showed a surface that looked very similar to the surface of Earth’s Moon.13 Mariner 10 measured a small magnetic field that was about one sixth as strong as Earth’s magnetic field.18 The presence of the magnetic field was important because scientists had expected that the core would be solid, and that Mercury would not have a magnetic field. The most likely source of the planet’s magnetism would be a molten metallic (iron) core. A molten core that was active would mean that the planet would also be tectonically active, and would still be cooling. The existence of a magnetic field on Mercury contrasted sharply with the Moon, where no global field could be detected, and was a strong indication that the formation of Mercury was different from the formation of the Moon.18 The planet’s composition was not well known after the mission, but it was estimated to have a content of 0 to 3-4% iron oxide (FeO) and to have an anthracitic crust.18 The images that were produced during the Mariner 10 mission showed six main categories of geologic features on the planet’s surface: (1) a widespread unit, intercrater plains, (2) heavily cratered plains, (3) the Caloris basin and related deposits, (4) smooth plains, (5) hilly and lineated terrain antipodal to the Caloris basin, and (6) numerous younger craters and their related deposits.14
Scientists did not expect to find a surface that had undergone hemispheric-scale crustal deformation. The main display of this deformation was crustal shortening, which was confirmed with the photographs taken by the Mariner.14 They were also surprised by the presence of many landforms created by tectonic processes, including smooth and intercrater plains that were spread over the Caloris basin in the highland and lowland plains.14 The images of the planet looked bleak and similar to the Moon, with the major difference being the presence of crustal shortening tectonic activity, presented in the form of scarps on Mercury’s surface.18
The high density of Mercury was another puzzling question to astronomers; how could such a small planet be so dense? The most widely accepted hypothesis was that the density must be due to a high concentration of metals.19 But that hypothesis brought with it more questions; what is the exact composition, and how was the core put together in the early stages of the formation of the planet?18 These questions would remain unanswered for another 30 years until the launch of the MESSENGER mission.
3.4. Results
The main findings of the mission were that the surface has been heavily bombarded and underwent crustal shortening. It was also calculated from data collected that the metallic core of the planet has a radius of 1800-1900 km, which makes up around 85% of the planet’s total radius.18 Scientists also concluded that the core was molten rather than solid, partly from telescope observations and the presence of a magnetic field that was detected by Mariner 10.18
The Mariner 10 mission was the first to visit Mercury, and also the first to change its trajectory using another planet’s gravity.13 The findings of Mariner 10 showed that the planet was quite similar to the Moon in terms of the surface and the lack of atmosphere. The largest difference, and perhaps surprise, to scientists was that the planet had undergone a lot of crustal deformation, most likely from the cooling of the planet.18 Although Mariner 10 provided a lot of insight to the mysterious and unexplored planet it also left scientists with more questions and curiosity than before the mission. The unanswered questions would be the basis for the MESSENGER mission that was launched nearly 30 years later.
4.0 Radar Suggests the Presence of Ice
Between the completion of the Mariner 10 mission and the launch of MESSENGER, significant new findings pertaining to Mercury’s geology were few and far between. Nevertheless, a number of developments occurred in the 1990’s using ground-based telescopes, hinting at exciting possibilities on the planet’s surface. As previously noted, because Mercury is only ever visible near the horizon, light reflected off its surface must travel through a long air path, which has historically hindered visible light observations. However, the recent development of adaptive optics technology provides a solution to these atmospheric effects. Towards the end of the decade, the power of adaptive optics gave astronomers their first look at the hemisphere of Mercury which Mariner 10 had been unable to image. In 1998, researchers utilized a technique known as selective image reconstruction (SIR) at the Mount Wilson Observatory, and were thereby able to provide the first high-resolution images of areas that had hitherto remained unphotographed.4 Several features were noted, including broad albedo variations, small bright spots, and a circular feature approximately 150 kilometres in length, which was hypothesized to be impact-related.4
At the same time, radio telescopes provided an alternative, simpler solution to atmospheric effects. Because radio waves penetrate Earth’s atmosphere and are not scattered like visible light, observations at this wavelength are undistorted and possible throughout the daytime. Radio telescopes have therefore provided important information about Mercury’s surface in recent years. Most significantly, observations using radar in the 1990’s suggested the presence of ice near the poles of the planet. Two telescopes were critical to this discovery: the NASA Deep Space Network 70-m Goldstone dish antenna working in conjunction with 26 Very Large Array (VLA) antennas, and the Arecibo radio telescope.20 In 1991, a Goldstone/VLA study detected an anonymously bright feature located at the north pole.21 The reflected signals from this area were also depolarized, which is a typical effect of ice, but not of the silicate rocks that compose the majority of Mercury’s surface.20 The same year, the Arecibo telescope also detected the bright spot, as well as an additional feature at the South Pole.21 Subsequent analysis of the Arecibo data revealed that these bright areas were located in crater-sized spots.21 It was hypothesized that the floors of craters located near the poles could provide a permanently shaded environment, explaining how ice could exist on a planet so close to the Sun.20 After an upgrade to the Arecibo telescope, higher resolution images were taken in the summers of 1998 and 1999, making an even more compelling case for the existence of ice (Figure 6).21 However, just as the topography of Mercury remained uncertain until the Mariner 10 mission, the presence of ice could not be definitively determined until a new spacecraft could be sent to the planet.22 The images taken by Mariner 10 could not be used to support or disprove the ice hypothesis, because they did not penetrate the dark interiors of the shadowed craters.20 Thus, these radar studies could provide no definitive answers, only intriguing possibilities which demanded closer investigation. That demand was finally answered with the arrival of MESSENGER.
5.0 MESSENGER Mission
5.1 MESSENGER Mission: Overview
In 2004, MESSENGER was sent off toward Mercury to gather information about the densest and least explored terrestrial planet.23 To plan the MESSENGER mission’s route, scientists included calculations which considered the gravitational pull of Venus, because it would greatly augment the rocket’s capabilities and ability to guide the spacecraft into Mercury’s orbit,24 creating a “slingshot” effect. MESSENGER took many photos with multiple instruments in order to study the atmospheric, topographic, and geologic makeup of Mercury. Thanks to the MESSENGER mission, identification of elements within Mercury’s crust took place, and it was confirmed that Mercury is capable of housing ice.23 After having orbited Mercury for four years, acquiring images of slightly more than half the planet, MESSENGER completed its mission at the end of April 2015.23
5.2 MESSENGER Mission: About the Ship/Instruments Used
MESSENGER’s many data collecting instruments were attached to the spacecraft quite rigidly, requiring MESSENGER to adjust its position to capture a reading. It used a main bipropellant engine for large trajectory-correction maneuvers and 16 small monopropellant thrusters for small orbit corrections.25 MESSENGER’s power source came from its array of solar panels. Power was stored in a battery in order to be distributed to all MESSENGER’s devices and systems. The spacecraft was built with backup systems to replace any one system that may have failed, and its panels were programmed for rotation to avoid overheating.25 The spacecraft housed many instruments, including dual imaging systems, several spectrometers, an altimeter, a magnetometer and sophisticated radio technology, for the detection of material (Figure 7).
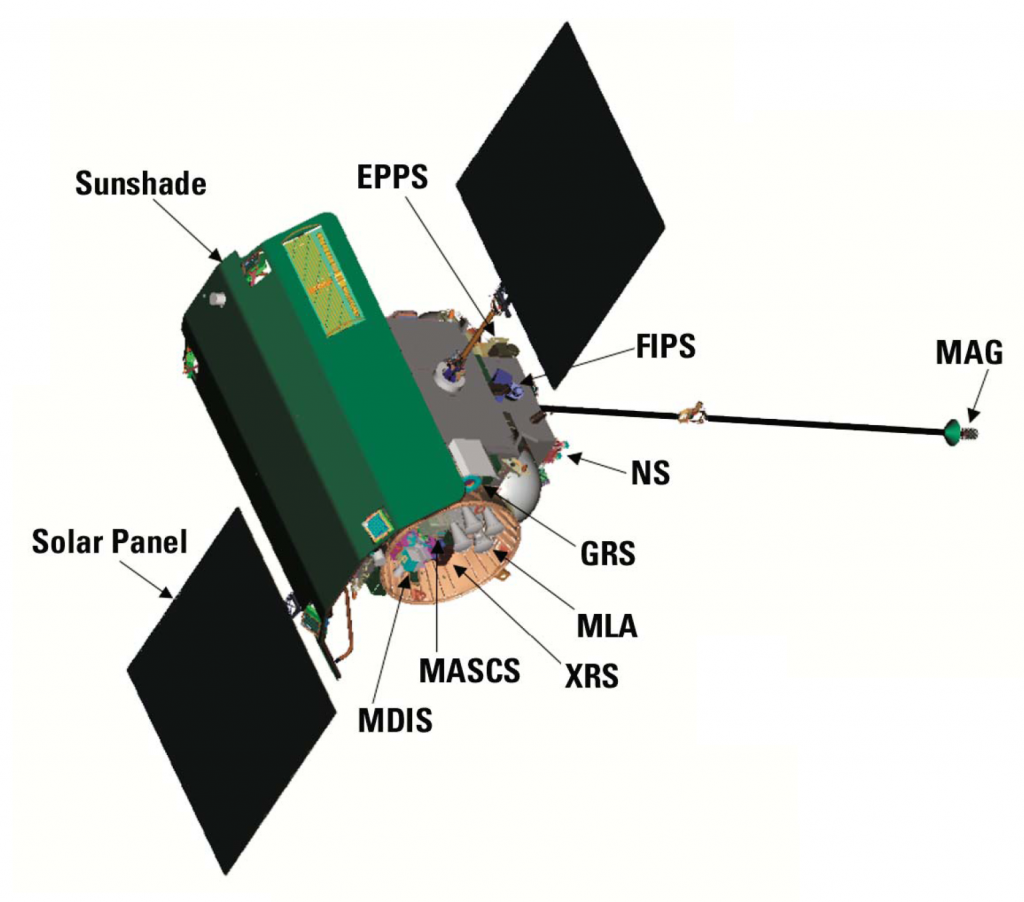
Figure 7: MESSENGER’s Science Payload. Credit: NASA/Johns Hopkins University/Applied Physics Laboratory
MESSENGER’s systems included the Mercury Dual Imaging System (MDIS), made of both wide and narrow-angle imagers. It gathered information about topography and surface spectra variations. These two imagers allowed MESSENGER to “see” similarly to two eyes.26 Gathering information about the reflection of light off Mercury’s surface allowed scientists to come to understand the chemical makeup of the surface itself. MESSENGER also contained a Gamma-Ray and Neutron Spectrometer (GRNS) which detected gamma rays and neutrons as they escaped radioactive elements on the surface of Mercury. The GRNS assisted in detecting the presence of ice at Mercury’s poles.26 Also upon the spacecraft was an X-Ray Spectrometer (XRS), used for detecting elements by reading X-rays produced as the Sun’s Gamma rays and X-rays hit Mercury’s surface.26 A sensor-like device called a Magnetometer (MAG) was used to detect Mercury’s magnetic fields and magnetized rocks within Mercury’s crust.26 MESSENGER also used a Mercury Laser Altimeter (MLA) to ascertain Mercury’s topographical information. Using a laser to measure differences in light reflection times, it was able to create a highly detailed depiction of Mercury’s surface.26 The Mercury Atmospheric and Surface Composition Spectrometer (MASCS) was used to detect light from infrared to ultraviolet spectra. It measured levels of atmospheric gasses and was also able to identify minerals on Mercury’s surface.26 Also included in the MESSENGER Mission was an Energetic Particle and Plasma Spectrometer (EPPS), responsible for observing charged particles such as electrons and ions in Mercury’s magnetosphere. The EPPS recorded information about the particles’ chemical makeup and composition, their distribution and their energy potential.26 MESSENGER’s Radio Science (RS) device measured shifts in frequency pertaining to the distance between a sound source and its observer to determine changes in the spacecraft’s velocity while in orbit.
5.3 MESSENGER Mission: Spectrometry
Before MESSENGER’s mission, scientists were unsure of the elements present on the planet’s surface. While Mercury’s bulk density had been determined, specific minerals had yet to be detected.27 The GRNS, EPPS, MASCS and XRS were all spectrometers specifically designed to uncover what Mercury was actually made of. Spectrometers detect data about light emitted from elements within the surface, atmosphere, and the gasses between, when they are excited by radiation (often from the Sun). Each element responds to spectra differently, allowing scientists to accurately identify chemical compositions of surface materials.27
5.4 MESSENGER Mission: Surface Findings
An onboard ultraviolet spectrometer was used to collect information on the radiation in the upper atmosphere. It saw ultraviolet radiation through a photometer and an imaging mass spectrometer. This showed oxygen, hydrogen, helium, potassium, water vapor, and silicon in Mercury’s atmosphere.23
Exploring Caloris, one of Mercury’s largest craters, the XRS was able to determine the presence of magnesium, silicon, calcium and aluminum in various compounds, suggesting the material might be similar to an alkali-basalt.26
XRS was only able to detect the composition of the top 1 millimeter of the surface, therefore analyzing a crater such as Caloris was useful as deeper layers of material would likely be exposed at least partially. The XRS and GRS were able to produce map of the surface chemistry content (Figure 8), detailing the location of certain elements composing Mercury’s terrain.28

Figure 8: Maps of magnesium/silicon (left) and thermal neutron absorption (right) across Mercury’s surface taken during the MESSENGER mission. Credit: NASA
As it was a subject of interest to Mercury researchers, several small-area, high-resolution images were taken to get a closer look at Mercury’s “volcanic snow”. Scientists investigated a group of volcanic vents with a camera much more powerful than the one that had been used during a preliminary investigation in 2009, allowing them to collect highly detailed images of pyroclastic dusting across surrounding craters, “softening” their appearance.29
One of the primary goals of the MESSENGER mission was to identify unusual materials at the poles of Mercury. As discussed earlier, observers had been able to see distinctly reflective regions at the poles of Mercury, hidden within cratered, permanently shadowed areas, hinting at the presence of water ice.30 Although Mercury is the planet closest to our Sun, reaching temperatures of extreme heat (800⁰F) in areas of exposure, the lack of atmosphere prevents the Sun’s heat from becoming trapped, allowing for extreme cold (-290⁰F) in shaded areas.
Through variable-adjusted simulations, observations, comparisons and studies, a team of researchers attempted to identify the presence of water ice on Mercury by analyzing the measurements collected by MESSENGER’s neutron spectrometer (GRNS). They found changes in the neutrons present in Mercury’s north polar region, indicative of water ice presence in the permanently shadowed areas.31 Data collected on the radar-bright polar region revealed it contained, on average, a layer more than tens of centimetres thick with hydrogen-rich properties, beneath the less hydrogen-dense surface.31 The team chose to attempt identification of the hydrogen-rich material by comparing simulations with the data collected by MESSENGER. The best simulation-to-data match indicated the buried hydrogen-rich material was very likely pure water ice.31 By measuring radar-bright areas, inferring ice depth and thickness based on observations from shifting landscape features (such as burial or disinterring caused by impacts and eruptions), it was estimated that Mercury contains 2 x 1016 to 1018 grams of water ice, however the team admitted that if the water ice layer is thicker than they calculated, this number could be larger.31
By combining analyses from Earth-based radar readings, Mercury’s topography, magnetic field and gravity field, clues as to what composed Mercury’s core have been revealed. Because the planet’s rotation seemed unusual, it was questioned whether Mercury’s core was solid and adhered to its mantle, or if the planet’s unusual spin was due rather to a molten core. The latter theory would allow the mantle to rotate freely, in a different manner than the core itself.30 It was determined that about 85% of Mercury’s planetary radius belongs to its core. Assumptions that Mercury’s core is at least partially liquid were supported by radar, gravity field and magnetic field observations.32
6.0 Conclusion
From our research we were able to summarize the findings of telescope observations, the Mariner 10 mission, radar results, and the MESSENGER mission and what each one found and how it contributed to our understanding of the planet, our Solar System, and our own planet. Knowing the geologic and atmospheric composition of other planets gives scientists insight into how our Solar System was formed and how it is currently behaving. Ground-based observations of Mercury are difficult because of the close proximity to the Sun, but scientists were nevertheless able to determine that Mercury is very dense and that it has a surface that resembles Earth’s Moon.6 Mariner 10 was the first mission to visit Mercury; it orbited the planet 4,000 times and was able to photograph approximately 45% of the surface.33 It confirmed the surface is similar to the Moon, and the surface has been heavily bombarded and has experienced crustal shortening.13 The mission provided a lot of answers, but left scientists with many questions that would be the basis for the MESSENGER mission that launched around 30 years later. The MESSENGER mission was launched in 2004 and concluded in 2015 when it crashed into the surface of Mercury.33 From that mission some of the most important findings were: (1) It showed that oxygen, hydrogen, helium, potassium, water vapor, and silicon are present in Mercury’s atmosphere,24 (2) It confirmed that there is snow (water ice) at the poles of the planet,32 (3) It confirmed that Mercury has a very large, dense, metallic core and (4) In the Caloris Basin the spacecraft found magnesium, silicon, calcium and aluminum in various compounds, suggesting material that might be similar to an alkali-basalt.27 The main findings over the course of two missions and telescope observations were the chemical makeup of the planet, the composition and consistency of the core, the geology, and formation history of the planet.
The story of Mercury’s exploration in the past half-century is one of both confirmed predictions and startling surprises. Mercury’s cratered surface, its high density, and its basic mineralogy were all initially predicted from telescopic observations, and these characteristics were subsequently confirmed and explored in far greater depth by the Mariner 10 and MESSENGER probes. Along the way, several unexpected discoveries have been made, such as Mariner 10’s detection of a global magnetic field, and the revelation that water ice is located at the poles. Learning about the swift planet has led to more informed hypotheses of Solar System formation and has enabled astronomers to better engage in comparative planetology. For example, because Mercury exhibits a magnetic field despite its slow rotation, astronomers know that fast rotation is not always an important factor in magnetic field generation, an insight which cannot be easily obtained from observing the other planets. Furthermore, the presence of ice on Mercury, a planet which exhibits surface temperatures in excess of 700 K, has expanded astronomers’ perspectives of where ice can form. This finding also serves as an important reminder that diverse environments can exist on seemingly uniform planets. Although the diminutive Mercury can sometimes be neglected amongst its larger terrestrial brothers, the insights it has provided into the processes at work in the inner Solar System, and Earth’s place within that framework, have been invaluable.
References
1 NASA, NASA Completes MESSENGER Mission with Expected Impact on Mercury’s Surface, WWW Document, (https://www.nasa.gov/press-release/nasa-completes-messenger-mission-with-expected-impact-on-mercurys-surface).
2 NASA, New Discoveries at Mercury, WWW Document, (https://science.nasa.gov/science-news/science-at-nasa/2008/03jul_mercuryupdate).
3 NASA, Messenger | All About That Basin, WWW Document, (https://www.nasa.gov/image-feature/tectonically-active-planet-mercury).
4 R.F. Dantowitz, S.W. Teare, and M.J. Kozubal, The Astronomical J. 119 (5), 2455 (2000).
5 N. Divine, The Planet Mercury (NASA, 1972), pp. 2-15.
6 R. Greeley and R. Batson, The Compact NASA Atlas of the Solar System (University Press, Cambridge, 2001), p. 39.
7 M.E. Davies, S.E. Dwornik, D.E. Gault, and R.G. Strom, Atlas of Mercury (NASA, 1978), pp. 2-5.
8 European Southern Observatory, Mercury Transit on May, 7, 2003, WWW Document, (https://www.eso.org/public/outreach/eduoff/vt-2004//mt-2003/mt-mercury-mapping.html).
9 F.W. Price, The Planet Observer’s Handbook, 2nd Ed. (University Press, Cambridge, 2000), pp. 75-79.
10 M.E. Davies, S.E. Dwornik, D.E. Gault, and R.G. Strom, Atlas of Mercury (NASA, 1978) pp.14-16.
11 B. Dunford, Dueling Desolations: Mercury and the Moon, WWW Document, (http://www.planetary.org/blogs/guest-blogs/bill-dunford/20130513-duelling-desolations-mercury.html).
12 M.A. Seeds and D.E. Backman, The Solar System, 8th Ed. (Brooks/Cole, Boston, MA, 2013) pp. 465.
13 NASA Solar System Exploration, Mariner 10 | Missions | Mariner 10: In Depth. WWW Document, (http://solarsystem.nasa.gov/missions/mariner10/indepth).
14 J.W. Head et al., Space Sci. Rev. 131 (1), 41 (2007).
15 NASA, Mariner 10 Mission And Spacecraft: SP-423 Atlas of Mercury, WWW Document, (https://history.nasa.gov/SP-423/mariner.htm).
16 R.G. Strom and A.L. Sprague, Exploring Mercury: The Iron Planet (Praxis Publishing Ltd, UK, 2003), pp. 15-33.
17 NASA Solar System Exploration, Mariner 10 | Missions | Mariner 10: Galleries, WWW Document, (http://solarsystem.nasa.gov/missions/mariner10/galleries).
18 E. Howell, Mariner 10: First Mission to Mercury, WWW Document, (http://www.space.com/18301-mariner-10.html).
19 B.W. Denevi, M.S. Robinson, Icarus 197 (1), 239 (2008).
20 D.R. Williams, Ice on Mercury: The Pre-MESSENGER Picture, WWW Document, (http://nssdc.gsfc.nasa.gov/planetary/ice/ice_mercury.html).
21 J.K. Harmon, Mercury Radar Imaging at Arecibo, EGS XXVII General Assembly (2002).
22 NASA, Water Ice on Mercury, WWW Document, (https://www.nasa.gov/image-feature/water-ice-on-mercury).
23 T. Talbert, NASA | MESSENGER Overview, WWW Document, (https://www.nasa.gov/mission_pages/messenger/main/index.html).
24 C.R. Chapman, Science Scope 27 (6), 40 (2004).
25 T. Talbert, NASA | MESSENGER: The Extreme Machine, WWW Document, (https://www.nasa.gov/missions/solarsystem/f_messenger-extreme.html).
26 T. Talbert, NASA | MESSENGER Spacecraft and Instruments, WWW Document, (https://www.nasa.gov/mission_pages/messenger/spacecraft/index.html).
27 P. D’Incecco et al., Planetary and Space Science 119, 250 (2015).
28 T. Talbert, NASA | MESSENGER Surface Chemistry Maps, WWW Document, (https://www.nasa.gov/image-feature/surface-chemistry-maps).
29 T. Talbert, NASA | MESSENGER Volcanic Snow, WWW Document, (https://www.nasa.gov/image-feature/volcanic-snow).
30 V. Jaggard, National Geographic News | Mercury Messenger 101, WWW Document, (http://voices.nationalgeographic.com/2011/03/14/nasa_mercury_messenger_enter_orbit/).
31 D. Lawrence et al., Science 339, 292 (2013).
32 Space Daily, MESSENGER Provides New Look at Mercury’s Landscape, Metallic Core, and Polar Shadows, WWW Document, (http://go.galegroup.com.cyber.usask.ca/ps/i. do?p=ITOF&sw=w&u=usaskmain&v=2.1&it=r&id=GALE%7CA285168824&asid=3e4069de0385a7af79249a65 ce4b78f6).
33 I. Semeniuk, NASA’s Messenger mission set to end, will crash into Mercury’s surface, WWW Document, (http://www.theglobeandmail.com/technology/science/nasas-messenger-mission-set-to-end-will-crash-into-mercurys-surface/article24177993/).
34 Dictionary.com, WWW Document, (http://www.dictionary.com/).
35 Geology.com – Dictionary, WWW Document, (http://geology.com/geology-dictionary.shtml).