Mars
Nicholas Brunoro, Lex Edmunds, Jasmine Redford, Kaitlin Phaneuf, Brydon Wandzura, Samantha Whittingham
Properties of Mars
Mars is the fourth planet from the sun at 1.523679 AU. The red planet is Earth’s closest neighbour, and has garnered speculation regarding colonisation as far back as the seventeenth century, perhaps most notably in H. G. Wells’ War of the Worlds. 1
“Mars Fever” hit its zenith in the science-fiction renaissance of the 1950s with such literature and films as: pop-culture embracing spherical bio-domes amidst bleak red Martian deserts, pulp-novel Martian adventures, literary accomplishments such as Ray Bradbury’s: The Martian Chronicles and movies where Mars’ “little green men” greet humankind with open arms or set about invading Earth themselves. Interest regarding the Mars migration is growing steadily; it is more important than ever to consider the feasibility of what was once merely science fiction, especially examining the possibility of terraforming our neighbouring planet so that it may sustain life as we know it on Earth—animal, vegetable, and mineral.
The possibility of terraforming Mars has become an increasingly popular topic of speculation among esteemed scientists. Stephen Hawking has adjusted his prediction that human survival depends on the colonisation of another planet within the next 1 000 years, to the next 100 due to factors such as over-population and pollution.2
The Challenges of Living on Mars
Earth and Mars, the only two planets located in the halo-shaped region around the Sun called the “habitable zone,” are the only astronomical objects in our solar system theoretically capable of housing liquid water. Liquid water is essential to life, NASA astrobiologist Chris McKay has gone as far as to say that water is the “single non-negotiable thing life requires”.3
Mars is situated on the edge of the “habitable zone”, so while is has the potential to form liquid water, we have yet to see surface-water. While NASA has confirmed the existence of ice deposits on Mars, we have yet to find surface-water.4
There are many aspects of life on Mars that will need to be transformed for human life to be possible on Mars. Atmospheric pressure, for instance, presents the problem of a planet with a surface pressure equivalent to “an altitude of 35 km above sea level on Earth, far higher than Earth’s highest mountain”.5 Mars contains some water vapour in the atmosphere, but due to the planet’s chilly temperature, this vapour often resolves itself as ice clouds or ice fog.5 Unfortunately, for want of plants, Mars has only a minuscule amount of oxygen; this is a fairly pressing issue if the planet is to sustain life.
Like Venus, the atmosphere of Mars is composed almost entirely of carbon dioxide, 95%, and a lesser amount of nitrogen 2.7%.5 The near absence of oxygen means that Mars has very little ozone. Without ozone, solar UV radiation reaches all the way to the surface. These UV rays could be lethal to any surface life-forms, so any life would either need to develop protective layers or be located away from direct exposure on the surface of the planet; for example, in caves or below the surface.5
All this considered, the red planet itself has living conditions relatively equivalent to extreme Earth conditions—potentially, if not currently, habitable. There are obstacles but, with time and resources, they may be overcome. The condition of the atmosphere on Mars is problematic, but there are potential solutions, such as living below the surface. These initial solutions, while relatively undesirable, present a starting point for more potentially desirable conditions as technology continues to advance.
Using Shallow Radar (SHARAD), NASA reports that—as of 2016—Mars potentially holds as much water as Lake Superior.6 These previously suspected, now confirmed, suspicions do much to bolster terraforming optimism.
Scientists examined part of Mars’ Utopia Planitia region, in the mid-northern latitudes, with the orbiter’s ground-penetrating Shallow Radar (SHARAD) instrument.6 Analyses of data from more than 600 overhead passes with the on-board radar instrument revealed a deposit more extensive in area than the state of New Mexico. The deposit ranges in thickness from about 260 feet (80 meters) to about 560 feet (170 meters), with a composition that’s 50 to 85 percent water ice, mixed with dust or larger rocky particles.6
Mars’ ice caps are sources of water, albeit in solid frozen form. In 2001, Odyssey confirmed the existence of water ice in the arctic regions of Mars using its Gamma Ray Spectrometer Instrument.7 However, the poles are not made solely of water. Frozen carbon dioxide, also known as dry ice, is found in these regions as well. Today, much of this buried ice, considered by forward-thinking scientists as a “future resource,” exists at the Martian poles due to the planet’s current tilt of 25 degrees.8 For this reason, Mars has seasons just like Earth. In the north, this dry ice melts periodically during Martian summers, and freezes again during Martian winters. The South on the other hand is cold enough year round to always have a layer of dry ice around 8 meters thick.8
Terraforming Mars
To be able to terraform our neighbouring planet, Mars, it will need to have: a warmer temperature, more oxygen in its atmosphere, accessible liquid water, less atmospheric pressure, and a surface protected from UV radiation.
Terraforming Mars would require three major changes to overcome the environment: 1) building up the magnetosphere; 2) building up the atmosphere and; 3) raising the temperature. Mars’ low surface pressure with high carbon dioxide content would require altering. If Mars were heated, the carbon dioxide may help keep this thermal energy near the surface. However, the heat would create an adverse effect by melting the permafrost located on both poles and enhance the greenhouse effect.
Earth is bombarded by Solar wind every day and Mars is no different. The difference is that Earth has what we call a magnetosphere to protect us from most of the particles that hit it. This magnetosphere is formed by Earth’s magnetic field and its interactions with the solar wind.9 Mars does not have this due to its small magnetic moments.
It is believed that Mars did have a magnetosphere around 4 billion years ago, but it suddenly disappeared. Without its magnetosphere Mars’ atmosphere deteriorated over the years. Something akin to a magnetosphere is needed in order for the atmosphere to be built up again. NASA is continuing to develop that something.
Rebuilding a Magnetosphere
One proposal is to not rebuild Mars’ magnetosphere at all, but instead put a magnetic shield in between Mars and the Sun at Mars’ L1 Lagrange Point. This artificial magnetosphere would deflect the solar wind coming from the Sun and allow the rebirth of a stable Mars’ atmosphere. In fact, just with this process alone NASA believes that Mars’ atmosphere would thrive again in time due to natural Volcanic out-gassing coming from Mars’ interior and Crust. This proposal was tested and confirmed in simulations at the Coordinated Community Modeling Center (CCMC). The CCMC is known for its specialty in space weather and the simulation was done by scientists from the Ames Research Center, the Goddard Space Flight Center, the University of Colorado, Princeton University, and the Rutherford Appleton Laboratory.
Another theory is to do something similar as fixing the magnetosphere. Instead of actually doing something on the surface of Mars, the Concentrated Sunlight Theory proposes the installation of mirrors to heat Mars.
Concentrated Sunlight Theory
By balancing gravitational and light pressure forces; Zubrin and McKay determined that a 125 km-diameter solar sail-mirror could be stationed 214,000 km behind Mars where it could illuminate the south pole with an additional – 27 TW.10 This should be sufficient to raise the polar temperature by 5 K which, according to some models, should be sufficient for cap evaporation.10 This theory would be able to warm the planet, but the problem is getting the materials and building the huge mirrors in space. The mining of these materials–from either Mars or other space objects–is possible, but there is the problem of how to make and assemble these huge mirrors with no people.
The Greenhouse Effect
Currently, the best theory to warm the planet is to use greenhouse gases. Scientist Christopher McKay proposes that the Greenhouse effect is enough to create an atmosphere around Mars, which would also warm the planet.11 McKay proposes making in abundance what he describes as “CFC cocktails.” CFCs being chlorofluorocarbons.11 These are chemicals that have C-Cl bonds that usually degrade very slowly. On Mars, since there is no ozone, the chemicals are not protected, causing the bonds to be less stable and broken down in a matter of hours.10 This has caused the idea of greenhouse gases to turn into an expensive endeavor since the gases will need to be added to the atmosphere periodically. These projections have led to the research of genetically engineered bacterium to produce the desired CFC greenhouse gases as a metabolic by-product of its living processes.12
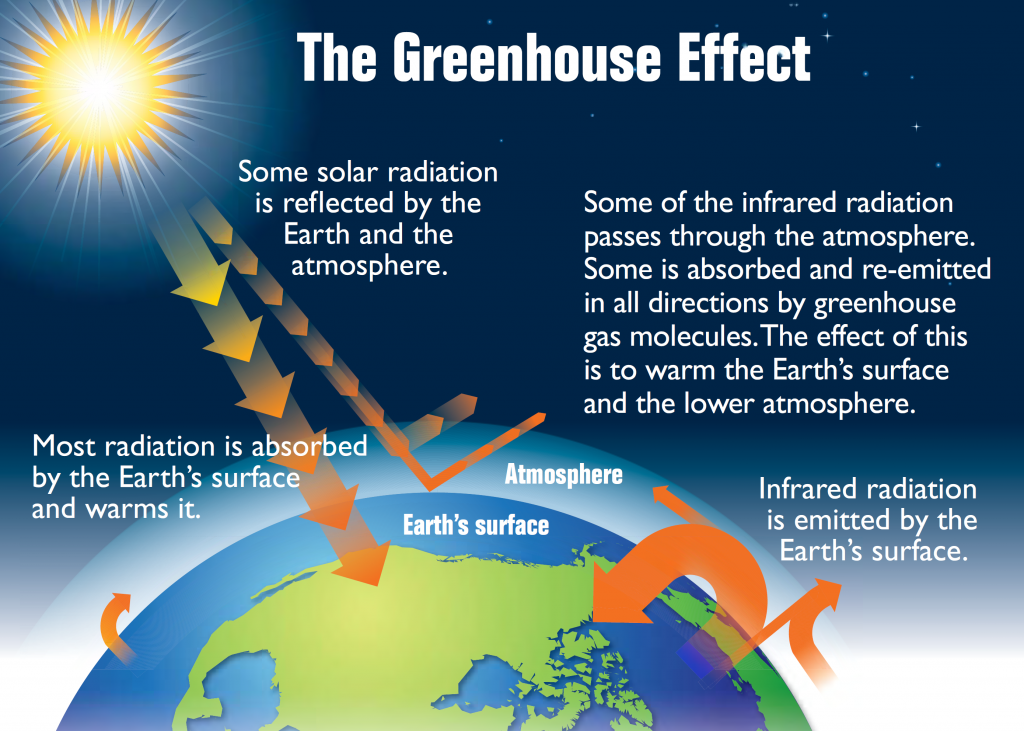
The Greenhouse effect on Earth, Mckay’s proposition would give Mars the atmosphere it needs to do this as well. Image Credit: US EPA [Public domain], via Wikimedia Commons
This may work, but more research needs to happen to transform the bacteria and make it survive in the harsh climate of Mars. These CFC cocktails would be made up of elements like carbon, nitrogen, oxygen, phosphorus, sulphur, and trace elements that have been detected on Mars. The most potent and long living CFC gases that cause a greenhouse effect, and whose elements are found easily on Mars, would be used; nothing that couldn’t be made from a scoop of Martian dirt would be made. Halon, a gas found in fire-extinguishers, is an example McKay mentions that would be easy to produce with Mars’ materials in abundance. The elements would be mined and the cocktails then produced from man made mining facilities on the surface. McKay estimates that at 750,000 metric tons of CFC gases a year Mars would warm up.11
Introducing Carbon Dioxide Into the Atmosphere
As the planet warms up, the soil and the ice caps would leak CO2 into the atmosphere. A chain reaction occurs, released CO2 would warm up the atmosphere more, and more CO2 would be released. “Since the North polar cap currently points away from the Sun at perihelion, a substantial amount of carbon dioxide ice could be stored there – up to an atmospheric pressure equivalent of 1 bar.”13
With the heating of the planet, the carbon dioxide that is trapped in the ice will be released into the atmosphere. The amount of carbon dioxide released from the ice is not substantial enough to change the atmosphere.13 On Earth, many chemicals are naturally stored underground in rocks. It is believed that there are large amounts of Carbonate in rocks that can’t be released by a slow warming as “devolatilisation of calcite requires temperatures of >1,000 K or shock pressures of > 100,000 bars.”13 Accessing these large amounts of carbon can be achieved by detonating a large number of thermonuclear explosives: “The intense shock wave from a nuclear explosion can de-volatilise carbonate minerals, producing a high pressure flow of gas away from the centre.”13 This means that thermonuclear mining may be the way to access water and minerals on Mars. There has been a discovery that the lowest regions of Mars may have aquifers. Pumps and drilling rigs would be required to access these possible aquifers, and with that help lowland lakes may be possible.13 With this greenhouse effect, McKay estimates that Mars would be warm enough for liquid water within a century.
With Mars now having an almost pure CO2 atmosphere, the next step is to make a green, oxygen filled Mars. Starting first with turning the CFC cocktail factories off, algae and plant seeds would be sent to Mars and planted. The plants would create oxygen and, over what McKay estimates at 100,000 years, the planet would become breathable for humans.12 However, it’s not that simple. Doing this would cool down the planet again. The factories would have to be turned on and off to keep a balance. A terraformed Mars though, does not have to be a Mars habitable for humans. A long time ago, Earth itself did not have an atmosphere fit for human life.
Conclusion
Currently life on Mars is not possible, and it is generally estimated that it would take at least 100 years to begin the process of making the planet habitable. Ultimately, to create life on Mars, the first thing that has to be addressed is the temperature. There are many solutions, but the truth is that it will take time for Mars to heat up to a temperature suitable for living. Once the temperature is raised it, will then start a chain reaction of thawing the poles and releasing the water trapped under the surface, which would then build up the atmosphere by releasing the gases trapped in the ground. All of these processes take a long time to achieve. After that then, the introduction of microorganisms becomes more of a possibility. Unfortunately, the move to Mars will take a while, and is unable to happen anytime soon with the current technology. Having said that, the following video allows us to imagine what mars would experience as it goes through a CGI rendition of the terraforming process.
References
- The Planets. 2017. <https://theplanets.org/mars/>
- J. Zorthian, TIME. (2017), <http://time.com/4767595/stephen-hawking-100-years-new-planet/>
- P. Bagne, Omni. 14 (10), 65 (1992). <go.galegroup.com/ps/i.do?p=EAIM&sw=w&u=usaskmain&v=2.1&id=GALE%7CA12297184&it=r&asid=5bf246de1a3c18b35ff13dd8a04fb951>
- https://www.nasa.gov/feature/jpl/mars-ice-deposit-holds-as-much-water-as-lake-superior FIX REFERENCE
- L. Kay, S. Palen, G. Blumenthal, 21st Century Astronomy, 5th ed., (W.W Norton, New York, 2016)
- T. Greicius, NASA. (2016). <https://www.nasa.gov/feature/jpl/mars-ice-deposit-holds-as-much-water-as-lake-superior>
- Phoenix Mars Mission. 1-6.<http://phoenix.lpl.arizona.edu/mars126.php>
- D. Darling. <http://www.daviddarling.info/encyclopedia/M/Marspoles.html>
- V. Badescu, Acta Astronautica. 56 (7), 670-680 (2005), doi:10.1016/j.actaastro.2004.11.004
- M. J. Fogg, Advances in Space Research. 22 (3), 415-420 (1998), doi:10.1016/S0273-1177(98)00166-5
- R. H. Haynes and C. P. McKay, Advances in Space Research. 12 (4), 133-140 (1992), doi:10.1016/0273-1177(92)90167-V
- R. L. S. Taylor, Advances in Space Research. 22 (3), 421-432 (1998), doi: 10.1016/S0273-1177(98)00167-7
- M. J. Fogg, Environmentalist. 13 (1), 7-17 (1993), doi:10.1007/BF01905499
- M. Williams, Universe Today. (2017), <https://www.universetoday.com/134052/nasa-proposes-magnetic-shield-protect-mars-atmosphere/>
- D.E. Canfield, Natural History. 122 (2), 16 (2014). <http://go.galegroup.com.cyber.usask.ca/ps/i.do?p=EAIM&sw=w&u=usaskmain&v=2.1&it=r&id=GALE%7CA362193801&asid=16f98335ece6d144014dedfb103efb63>